Hybrid Sol-Gel Coatings in Surface Engineering
A look at the use of modified sol-gel polymer films and hybrid system coatings, as well as the methodologies for evaluating the mechanical properties of the coatings.
Hybrid Sol-Gel Coatings in Surface Engineering: A Review
by
Xavier Albort Ventura*
Laboratory Electrochemical R&D, Barcelona, Spain
University Politecnic of Catalonia, Barcelona, Spain
Tecnocrom Industrial Cabrera de Mar, Barcelona, Spain
ABSTRACT
Sol-Gel chemistry offers a flexible approach to obtain a diverse range of materials. It allows different chemistries to be achieved and offers the ability to produce a wide range of nano-structures. Sol-gel materials have been explored and found to hold significant potential. One of the recent developments relates to hybrid materials that utilize sol-gel chemistry to achieve unusual composite properties. Sol-gel chemistry allows the combination of inorganic and organic materials in a single-phase and has led to the development of organic-inorganic hybrid (OIH) coatings for several applications, including corrosion mitigation. The advantages and disadvantages of using modified sol-gel polymer films and hybrid system coatings are discussed, as well as the methodologies for the chemical characterization and the feasibility of evaluating the mechanical properties of the coatings.
Editor’s Note: A printable pdf of this paper can be accessed and printed HERE.
Key Words and phrases: Sol-gel methods and chemistry; hybrid, corrosion metallic; polyelectrolyte multilayers; impedance in the layer-by-layer assembly; nanoparticles, polymers, layer-by-layer films; properties of nanoparticles and layer-by-layer polymeric assembly essential in building hybrid coatings; organic precursors in sol-gel methods; mechanism, the binary pore, layer-by-layer films; coatings, capsules.Microstructure and elemental characterization indicate that the finish of the layer-by-layer (LBL) coating consists of a closely connected multilayered coating with a smoother surface. The principles of assembly are also discussed together with the properties of nanoparticles and layer-by-layer polymeric assembly essential in building hybrid coatings.
1. Introduction
Aluminum and its alloys are widely used in the fields of engineering, aerospace and machine manufacturing because of their low density, low thermal expansion coefficients, high strength and strain performance, which have drawn much attention from both researchers and engineers as promising materials for electrical appliances and the machine industry. Under certain circumstances, Al alloys possess some anti-corrosion properties because of a naturally formed oxidized thin film. However, the naturally thin and inhomogeneous oxide film substantially dissolves when exposed to corrosive environments containing aggressive chloride ions, resulting in localized corrosion, mechanical failure, considerable financial cost and even catastrophic accidents.
There are two typical approaches to improve the corrosion resistance of Al alloys. One is to ameliorate the element proportion of Al alloys, and the other is to develop a protective film on the Al alloy surface.
Porous alumina films formed by the anodic oxidation of aluminum have been extensively studied for use as molds to form nanostructured materials. The technology of porous alumina and its usage as an anodic oxide coating in tools has a long history.
There is a great demand for the use of highly ordered nanohole arrays, which can be produced on a scale of several tens of nanometers through self-organization, in a diversity of applications. These include high-density storage media, functional nanomaterials exhibiting quantum size effects, highly sensitive chemical sensors, nano-electronic devices and functional biochemical membranes. Porous alumina membranes of anodic aluminum oxide (AAO) are widely used for the fabrication of various nanostructures and nano-devices. Over the last decade, many materials, including nanowires, nanotubes and nanodot arrays, have been fabricated by the deposition of various metals, semiconductors, oxides and polymers inside the pores of AAO
membranes.
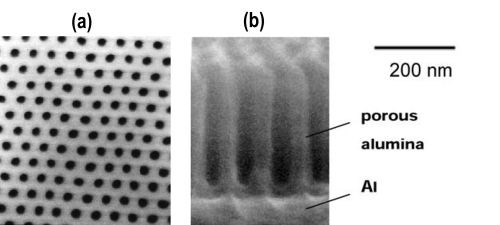
Figure 1 - SEM micrographs of an alumina nanohole array formed by two-step anodic oxidation at 40 V using 0.15M oxalic acid: (a) plan-view, (b) cross-sectional view (Shingubara, et al., 1997).
Nanoporous substrates, such as porous silicon, nano-porous anodic alumina, titania nanotube arrays and track-etched porous polymer membranes, have been commonly employed as substrates for advanced sensing devices. Nanoporous anodic alumina (NAA) processes produce unique structural, chemical, optical, thermal and mechanical properties, and biocompatibility, in addition to controllable geometry and exploitable surfaces chemistries.
Research has led to the production of organic-inorganic hybrid (OIH) materials with applications in a wide range of application, such as chemical and biosensors in several transduction modes, from electrochemical to optical, biomaterials for drug delivery, materials for improving bioactivity and biocompatibility of metallic substrates, electronics, environment, optics, functional medical smart coatings, fuel and solar cells, and catalysts (Fig. 2).
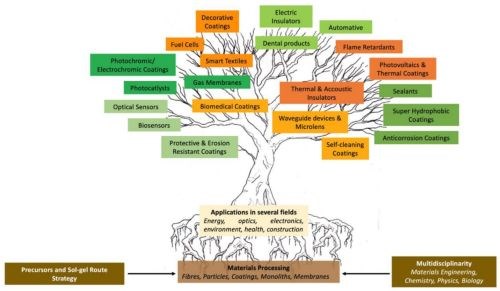
Figure 2 - Arborescence representation of IOH materials applications [from R.B. Figueira, “Hybrid Sol-gel Coatings for Corrosion Mitigation: A Critical Review,” Polymers (Basel), 12 (3), 689 (2020)].
However, such metallic alloys show some limitations, including low corrosion and wear resistance and unsuitable scratching and hardness properties. Therefore, in order to solve these drawbacks, several coatings have been proposed. Zirconium-based coatings and OIH materials have been widely studied and have been found to exhibit several enhanced properties; namely,
scratch resistance, biocompatibility, bioactivity and hardness.
Microporous organic polyimides (MOPI) Polymers
The sol-gel method offers several advantages when compared to traditional synthesis processes, namely the ease of fabrication and flexibility of the process, the large number of precursor reagents commercially available with tuned functional groups and low environmental impact. Furthermore, sol-gel allows for excellent control of the stoichiometry of the precursor, and the
incorporation of different components that introduce complementary functions to the material, as in Fig. 2, such as UV protection, anti-fouling, anti-reflection, moisture resistance, corrosion protection and the detection of biological components, proteins and enzymes, antibodies, DNA and polysaccharides.
Concepts of the sol-gel process
Development of the synthetic approaches to various oxide materials has been based on the polymerization of metal alkoxides M(OR)n, namely silicon, such as tetraethoxysilane (TEOS), or tetramethoxysilane (TMOS). This process is influenced by several factors, including pH, temperature, catalyst, solvent, nature of the alkyl group, water to alkoxide molar ratio, etc. Alkoxysilanes
generally show a slow kinetic rate when compared to the titanium, aluminum or molybdenum alkoxides. This allows better chemical control of the process, leading to materials with different properties, such as pore size and distribution, as well as particle dimension and shape. Furthermore, silicon shows lower reactivity regarding chelating groups and the redox process,
which may lead to the formation of sub-products.
Table 1 shows chemical structures of the most-used silicon alkoxide precursors in the synthesis of OIH materials. The precursors with polymerizable functional groups vinyl (VTMS) or methacrylate (MAPTS), besides allowing the formation of an organic network also contribute to further densification of the OIH material.
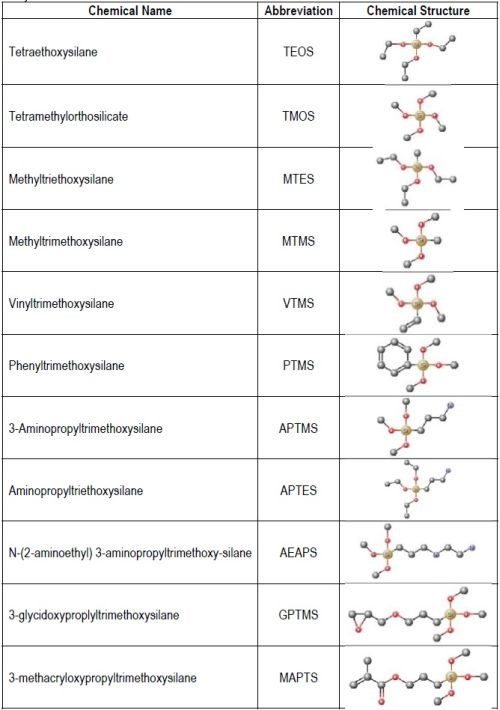
Table 1 - Abbreviation, chemical name and structure of each of the alkoxysilanes most used as precursors for synthesis of OIH materials.
Organic precursors in sol-gel methods
Silicon alkoxides represent the main network forming agents used in sol-gel preparation methods. While the sol-gel process provides key benefits, such as the low synthesis temperatures and the vast array of alkoxide precursors available, the cost associated with alkoxide precursors presents some limitations. Nevertheless, the efficiency provided by low-temperature
synthesis and the accuracy with which specific compositions can be achieved have the potential to outweigh any such negative aspects of the process. Low- temperature synthesis is achieved through solution-mediated formation of strong covalent bonds between elements that would otherwise require excessively high temperatures to create. For alkoxides this requires initial
hydrolysis of the alkoxy group followed by condensation between network forming substrates (Fig. 3).
Sol-gel methods also enable powderless processing of glasses, ceramics and thin films or fibers directly from solution.
Precursors are mixed at the molecular level and variously shaped materials may be formed at much lower temperatures than is possible by traditional preparation methods.
One of the major advantages of sol-gel processing is the ability to synthesize hybrid organic-inorganic materials. Combinations of inorganic and organic networks facilitate the design of new engineering materials with diverse properties for a wide range of applications. Biomedical applications invariably require the design of new biomaterials, and this can be achieved by emerging sol-gel chemistry and biochemistry. The gel-derived materials are excellent model systems for studying and controlling biochemical interactions within constrained matrices with enhanced bioactivity because of their surface chemistry, nanopores and large specific surface area. In biomedical applications, the coating of medical devices is an important issue.
Materials used in medical devices should have appropriate structural and mechanical properties and ideally promote a healing response without
causing adverse immune reactions. Medical services designers currently use various surface treatments such as coatings that enhance or modify properties such as lubricity, hydrophobicity, functionalization and biocompatibility. Sol-gel technology offers an alternative technique for producing bioactive surfaces for these applications.
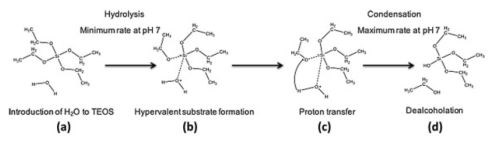
Figure 3 - Initial hydrolysis and condensation stages of TEOS in the production of silica oligomers: (a) Introduction of water to TEOS, (b) H2O forms a hypervalent substrate with silicon, (c) transfer of the proton from the water to the adjacent alkoxy group, (d) cleavage of the ester bond and dealcoholation.
Sol-gel thin film processing offers a number of advantages, including low-temperature processing, ease of fabrication and precise microstructural and chemical control. The sol-gel derived film or layer not only provides a good degree of biocompatibility, but also a high specific surface area and an external surface whose rich chemistry allows ease of functionalization by suitable biomolecules.
The development of multifunctional nanoparticles that can be used as drug delivery vectors remains a significant challenge for material science. These applications require intimate control of the particle size and discrete, superparamagnetic iron oxide nanoparticles that can be prepared by the sol-gel method to lower the annealing temperatures required.
Silica-based magnetic nanocomposites (MNP), formed by magnetic nanoparticles dispersed in a silica matrix, are of relevant technological and scientific interest. Here encapsulation in silica prevents interactions between the MNPs, and consequently assures a uniform dispersion. The latter is essential for efficient performance in most applications, including diagnostic and therapeutic uses, where particles must display high magnetization, be oxidation-resistant and most importantly, remain nonaggregated.
Engineering new bone tissue with cells and a synthetic extracellular matrix (ECM) represents a promising approach for the regeneration of mineralized tissues. Bone regeneration requires a scaffold material upon which cells can attach proliferate and differentiate into functionally and structurally appropriate tissues for the body location into which they are placed.
Bone is a highly mineralized tissue, consisting of an apatitic mineral phase most similar to a form of carbonated hydroxyapatite (HCA), although a significant contribution is made by extraneous ions such as sodium, chloride, zinc and, to a lesser extent, fluorides. In general, HCA can be considered a model mineral for natural bone and is widely accepted as a bioactive material with excellent biocompatibility, high osteoconductivity, and reasonable mechanical strength. For these reasons, it has been widely used in tissue engineering applications, especially for bone and cartilage regeneration.
However, in vivo data suggest that degradation or ion release from labile sources such as bioactive glasses promotes new bone formation, as opposed to the relatively lower ion release that occurs as a result of HCA minerals reaching equilibrium with their surrounding medium. It appears that sol-gel methods hold the potential to apply an ever-increasing range of glass-based
bioactive coating to materials, which have previously remained incompatible with alternative coating techniques. Furthermore, the versatility of the sol-gel approach is opening new doors to previously unattainable compositions, again increasing the potential applications of sol-gel materials as fillers to replace tissue within necrotic or defect sites.
Sol-gel microencapsulation technology and its broad application potential are now well-known. Relevant here is the use of silica for encapsulation and controlled release of hydrophilic molecules, ensuring considerable chemical and physical protection of the valued entrapped dopants. Given the above, it can be seen that sol-gel derived bioactive materials hold great potential value.
Sol-gel chemical and reaction process
The chemical reactions involved in the sol-gel process are usually divided into two steps. The first step involves the hydrolysis of metal alkoxides, producing hydroxyl groups in the presence of water.
The second step is followed by polycondensation of the resulting hydroxyl groups and residual alkoxyl groups, forming a three-dimensional (3D) network, establishing covalent bonds - Si-O-Si in the case of silicon alkoxides. Therefore, the alkoxides and esters of inorganic acids are raw materials of utmost importance in sol-gel technology. In the case of silicon alkoxides, the
hydrolysis and condensation reactions may be catalyzed by acids or bases.
The structure and morphology of the final material is strongly dependent on the nature of the catalyst and pH of the reaction. The hydrolysis reactions either catalyzed by acids or bases, are nucleophilic substitution reactions type SN2, characterized by a transition state in which the silicon atom is penta-coordinated. The replacement extension of the OR groups by OH groups in the silicon atoms depends on the molar ratio Si/H2O.
Si(OR)4 + nH2O Si(OR)4-n (OH)n + nROH
For acidic catalysis,the following reaction takes place (Reaction 1):

For basic catalysis, the following reaction takes place (Reaction 2):

The condensation reaction of Si(OR)4-n(OH)n may also be catalyzed by acids or bases. The reactions for acidic catalysis are (Reactions 3 and 4):
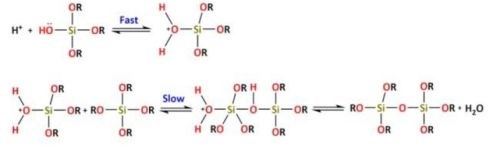
The reaction mechanism of condensation by acidic catalysis starts with the protonation of the silanol species ending with the formation of a Si–O–Si bond.
The reactions for alkaline catalysis are (Reactions 5 and 6):
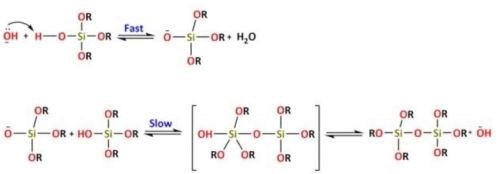
For alkaline catalysis, the specie HO-removes the proton from the silanol group, forming a silanolate anion ending with the formation of a Si-O-Si bond. The reactions of alkoxysilanes, in which monomers form polymeric structures by loss of substituents, involve the formation of a colloidal suspension followed by the polymerization that will lead to the formation of a 3D structure sol-gel.
For acid catalysis, the alkoxysilane hydrolysis rate shows higher kinetics when compared to basic catalysis. Therefore, the acidic catalysis leads to the formation of a highly branched polymeric structure, while alkaline catalysis leads to colloidal particles with linear chains or a ringed shape. Once the gel is formed, the hybrid network needs to be dried in order to remove the water,
alcohol and solvents that are formed during the hydrolysis/condensation reactions.
The drying step has an important role since, depending on the process used, different materials will be obtained. For instance, if the drying process takes place under normal conditions, a contraction of the gelled network occurs.
The dry gel, which is known as xerogel, may shrink between five to ten times in volume when compared to the gel before drying.
The shrinkage process includes the transport of the liquid through the pores of structure and contraction of the network. The xerogel is suitable for substrate catalysis and filters, among other applications, mainly due to its low porosity and large surface area.
Several studies have been reported concerning the use of xerogels in the field of filters and membranes and for carbon dioxide capture. If the drying of the gel occurs in wet supercritical conditions, the shrinkage will be minimal, and the resulting product is porous and is known as an aerogel. The material is known as a monolith if the dimensions of the gel are scarcely greater than
millimeters. In case of the gelation, it takes place by quick evaporation of the solvents. The materials obtained are coatings.
Nevertheless, it should be kept in mind that during the loss of the volatile by-products formed through the hydrolysis and condensation reactions, controlling the sample shrinkage during 3D network development may be challenging and puzzling. The type of material composed by organic and inorganic components and known as OIH, is extremely interesting, since it enables an interface between two worlds of chemistry (organic
and inorganic) each with unique properties that cannot be found on the
individual components.
Hybrid sol-gel coatings for corrosion mitigation
OIHs have numerous unique and desirable properties with application in a wide range of fields (Fig. 2 above). However, the considerable interest involved in the development of such materials is mainly due to the convenience of the synthesis process.
The sol-gel method is a flexible and simple route that allows one to obtain OIH materials in different forms (films, fibers, nanoparticles, etc.). The synthesis route is particularly valuable for the design and production of OIH coatings.
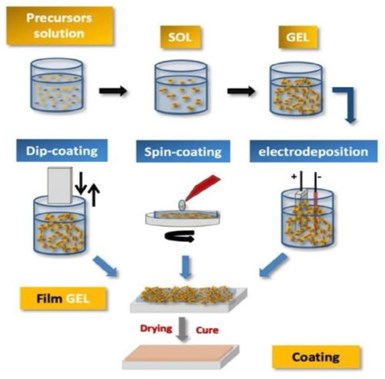
Figure 4 - Schematic diagram of steps and processes used to obtain sol-gel coatings.
Furthermore, the sol-gel synthesis route allows one to strictly control the precursor stoichiometry to modify and introduce functional groups or encapsulate different elements. Moreover, it allows one to coat substrates with large surface areas and minimum complex geometries involving simple and inexpensive equipment, as illustrated in Fig. 4. It is a process that involves the ability to manipulate nanostructural properties by controlling the hydrolysis, condensation reactions and aggregation processes.
The properties of OIH sol-gel materials such as homogeneity, stability and transparency, are largely dominated by the concentrations and types of precursors, the nature of the solvent, the concentrations and types of additives (surfactants, catalysts, etc.), the pH of the reaction media, the heat
treatment and the curing time. Furthermore, the OIH gel network can be modified with a vast number of dopants, leading to products with singular and extraordinary properties.
The requirements for a material to be used as a coating for corrosion mitigation must include effective protection against corrosion, abrasion resistance and a good adhesion between the coating and the substrate surface. The OIHs show properties such as high hydrophobicity, good corrosion protection, low dielectric constants and/or good scratch resistance.
Therefore, these chemical and physical properties make the OIH class the ones more suitable to mitigate corrosion oxidation of the metallic substrates. OIH class can be easily prepared by the sol-gel process in which the alkoxysilane precursor, generally a trialkoxysilane, has an alkyl group bonded to the silicon atom producing monomers in RSi(OR)3 in which R may be CH3, CH2-CH3 or an aromatic group. Within the alkyl group, by establishing a bond, the C-Si does not react and remains covalently bonded to the silicon groups through the C-Si bond, making part of the final material structure.
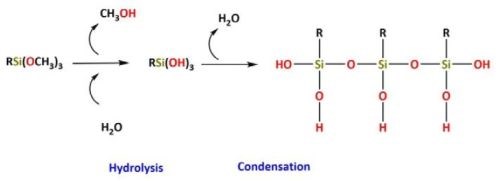
Figure 5 - Generic two-step reactions involved in the preparation of OIH materials through the sol-gel method using Si as the network-forming element. R is typically an alkyl group. The organic groups, through the combination of suitable monomers, may allow the formation of bridges between the silicate groups.
Metal chelation in the sol-gel
In aqueous solution, metal ions are coordinated by a hydration shell, the nature of which depends both on the valence of the specific metal in question and the pH of the solution. This ultimately results in the formation of polymeric oxides in solution.
With the formation of a hydroxy-ligand, a bridge is thus established between the two hydrated metal complexes. This results in the release of a proton into the aqueous medium and is followed by subsequent deprotonation event to form a resultant M-O-M covalent bond. From this brief description alone, the influence of pH on the process can also be deduced. An increase in pH
favors olation** while a lower pH inhibits the process.
Undoubtedly, materials composed of metal oxides exhibit a wide range of desirable properties and as a result, a series of methods have been developed, based on chelation of metal precursors, in order to control the natural polymerization processes.
Essentially, metal chelation sol-gel methods employ strong chelating agents (such as citric acid or EDTA) as a means of controlling the formation of the highly reactive hydrated complexes.
Although discussed here in terms of chelated inorganic precursors, chelation itself is not limited to inorganic processes. Such methods can also be applied to modify the polycondensation of metal alkoxides whereby the rate of reaction is reduced following the replacement of alkoxide leaving groups with a chelating ligand in more stable conformation.
In further discerning metal chelation methods from the alkoxide sol-gel route, the underlying principle is that polycondensation ofthe metal itself occurs through hydration processes described above, as opposed to the hydrolysis and condensation steps akin to the polymerization of organometallic precursors.
Based on a system that made use of triethanolamine as an Fe(II) chelating agent, triethanolamine is able to form chelation complexes with a wide range of transition metal elements, therefore offering a plausible route for further biologically relevant substitutions within the network.
The use of epoxides as gelation agents provides another useful synthesis pathway. Typically, epoxide routes are most effective when the formal oxidation state of the dopant cation is M3+, although species that possess a lower valence may also be incorporated. In this instance, the epoxide does not act as a precursor per se. Rather, the epoxide group is able to efficiently
accept protons, leading to the formation of hydrated oxo-ligands M(H2O)n(O)m-n)2+ coordination until deprotonation occurs in the
presence of an epoxide. Although not strictly a chelation-based method, parallels can be observed between this approach and the more typical chelation methods that aim to prevent natural polymerization processes until required.
Polymer-assisted sol-gel
In a natural extension from metal chelate methods, there are the polymeric sol-gel methods. Essentially these methods involve the chelation of reactive inorganic gel-forming agents within an organic polymer network although, depending on the material to be produced, chelation is secondary to the stabilization. In broader terms, gel-forming agents are maintained in a state of dispersion throughout the solution, thereby preventing the precipitation of aggregates within the sol. This method does however require subsequent heat treatment to remove the organic polymer following the formation of the inorganic gel.
Chemical properties, such as the biomimetic molar ratios of apatites, can also be achieved with polymer-assisted stabilization via the homogenous elemental distribution of the gel network.
Inorganic networks can also be formed in situ through the polymerization of organic precursors. This method involves the formation of a three-dimensional polyester network resulting from the reaction between ethylene glycol and citric acid. The citric acid acts as a chelation agent due to an available bi-dentate binding mechanism followed by an esterification with ethylene glycol (Fig. 6). The organic network would be removed as with ex situ polymers described earlier.
Effective production of both biocompatible ceramic-mineral composites and apatites with predefined stoichiometry has been achieved, with polymer-assisted sol-gel methods. Such research may also have broader implications in solving issuesassociated with biocompatibility as, with the advent of controlled deposition of inorganic mineral layers, abiotic (i.e., physical,
rather than biological) hard tissue regeneration may also be within reach.
____________________________________________________
**Olation is the process by which metal ions form polymeric oxides in aqueous solution.
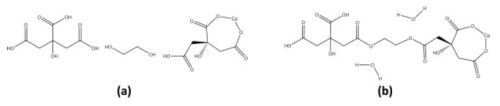
Figure 6 - Esterification (condensation) of ethylene glycol and citrate in the presence of a cationic ligand (calcium).
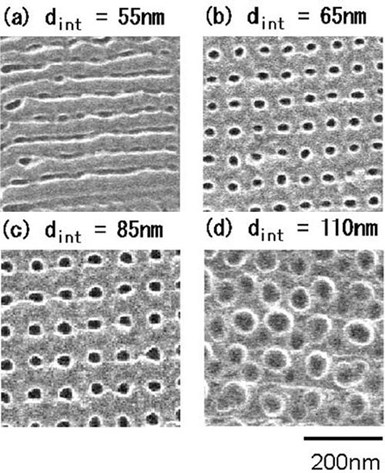
Figure 7 - Plan-view SEM micrographs of porous alumina film surfaces that were formed by AFM nano-indentation followed by anodic oxidation at 40 V using 0.15M oxalic acid for 5 min. Indentation force was 4.16 × 10−5N. Indentation interval dint was varied from 55 to 110 nm. (Shingubara, et al., 2002).
Silica-based sol-gel based materials
Silica-based sol-gel materials have been the subject of intense interest for the last three decades. Biomolecular encapsulation within sol-gel derived silica matrices was first successfully accomplished by entrapping enzymes into TEOS matrices.
During the last few decades, silica-based materials have supplied successful solutions for soft and hard tissue regeneration. These materials are highly biocompatible, and the positive biological effects of their reaction products
make them an interesting group of materials for tissue regeneration. Silica-based bioactive glasses were first synthesized via sol-gel techniques at lower processing temperatures compared to the melt-derived glasses.
Extensively researched sol-gel glasses are based on the SiO2-ONa-P2O3 system for biomedical applications. Silica-based sol-gel glasses exhibit many of the properties associated with an ideal material for tissue regeneration,
such as high surface area and a porous structure, in terms of overall porosity and pore size, that promote cell-material interactions and cell invasion. Research on these glasses has shown that the porous structure of these glasses yields a higher surface area that exhibits higher tissue bonding
rates.
A sol-gel process involving the foaming of a sol with the aid of a surfactant, followed by condensation and gelation reactions, has been used to prepare porous scaffolds of a few bioglasses, such as the glass designated with the
composition (mol%) 4O-SiO2-2O-ONa-2P2O3. The as-prepared scaffold had an overall microstructure similar to that of dry human trabecular bone, but the pore structure was hierarchical, consisting of interconnected macropores (<90 micron) resulting from the foaming process and mesopores that are inherent to the sol-gel process.
Figure 7 Illustrates the porous structure of the scaffolds made of bioactive glasses produced by means of sol-gel processes.
This hierarchical pore structure of the scaffolds is beneficial for stimulating interaction with cells as it mimics the hierarchical structure of many natural tissues and more closely simulates the physiological environment of mineralized tissues. Thanks to the nanopores in the glass, sol-gel derived scaffolds have a very high surface area (100-150 m2/g). As a result, these scaffolds degrade and convert faster to hard anodizing than those of melt-derived glass with the same composition. However, these solgel-derived scaffolds have a relatively low compressive strength, and consequently, they are primarily suitable for applications focused on low load bearing orthopedic sites.
The discovery of mesoporous silica nanoparticles was quickly recognized as a breakthrough that could lead to a variety of important applications. These materials have uniform cylindrical pores with a diameter size in the range of 2-20 nm, along with a large surface area between 500-1100 m2/g, making them ideal for chemical separations, catalysis and biomedical applications.
In general, solution synthesis is carried out under a basic condition, which is similar to the traditional method for preparation of silica particles.
Under basic conditions, a part of the silanol group Si-OH, is deprotonated to form silanate, Si-O. In order to match the negatively-charged silica surface, cationic surfactants are normally used. Thus, the hydrolysis and condensation rates of the silica sources are highly associated with several factors, such as pH, silica source, additives and temperature. The effect of pH
is critical as it affects nucleation and growth. These previous reports hint that minimum particle size can be obtained at around pH 9-10, indicating that the condensation rates, rather than the hydrolysis rate of silica precursors highly affect the final particle sizes.
There have been very few trials to prepare under acidic conditions. One of the advantages of acidic conditions is the ability to use block copolymers, which have a templating effect and allow larger mesopores, greater than 5 nm. The condensation rate for the synthesis at pH 5-8 should be faster than at pH values below 5. Since the condensation reaction solidifies the silica network, one can expect that a too rapid condensation reaction will create a cross-linked network faster than the mesostructure can organize.
This explanation is based on a formation mechanism where particle formation, silica condensation and ordering of the pore system are considered as separate processes. Thus, in order to obtain an ordered material, the rates of the different processes must be properly adjusted relative to each other. However, compared with basic synthetic systems, it is difficult to obtain a uniform particle size less than 90 nm and with spherical morphologies, but the large surface area of the pores allows them to be
filled with different biomolecules for biomedical applications.
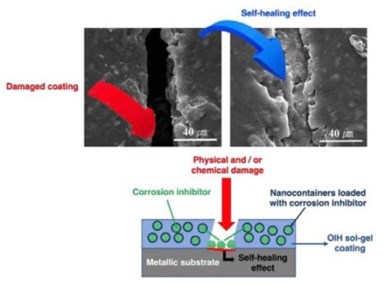
Figure 8 - Schematic diagram of the corrosion self-healing mechanism with an OIH sol-gel coating containing nano-capsules loaded with corrosion inhibitors.
Sol-gel coatings with a self-healing function
Self-healing ability can be achieved at different levels of the coating system
and by different strategies. Selfhealing is the ability of a coating, in a certain environment, to repair damaged areas through certain external means.
Self-healing coatings may be classified into two types: intrinsic and extrinsic.
Intrinsic coatings are those with a latent self-healing functionality and have the ability to heal the coating matrices by themselves. Extrinsic coatings have a healing agent microencapsulated and embedded within the coating matrix. In this case, when the microcapsules are ruptured by damage, pH change or
some other trigger, the healing agent is released and seals the damaged region, and the healing reaction takes place (Fig. 8).
Strategies, such as the addition of polymerizable agents, to restore/heal the damage in a polymeric coating or the inclusion of corrosion inhibitors, to stop the damage spread have been reported. Metals such as cerium, lanthanum or zirconium have been incorporated in OIH sol-gel coatings in order to stop corrosion propagation. Studies based on OIH coatings doped with cerium
inhibitors have also been reported.
Concerning the use of cerium as a corrosion inhibitor, a comparative study was performed on the effect of LaO3 nanoparticles (NPs) and La(III) ions as corrosion inhibitors in silica-alumina OIH sol-gel coatings. The coatings doped with lanthanum nitrate (La(NO3)3) showed poorer corrosion behavior when compared to LaO3 NPs. This behavior was not reported for coatings doped with LaO3 NPs. It was also concluded that the coatings doped with LaO3 NPs tended to further minimize the electrolyte ingress and therefore provided improved protection. The authors concluded that the curing procedure affected the coating properties, obtaining a coating with lower roughness, reduced thickness and higher density by using a curing heating ramp.
The incorporation of La(NO3)3 increased the roughness and thickness linked to a network with higher porosity. The electrochemical data indicated that the coatings doped with La(NO3)3 and cured with heating ramp exhibited improved corrosion properties. The effects of curing temperature and the addition of La(NO3)3 on the degree of polycondensation of the OIH sols
were studied. It was concluded that the addition of La(NO3)3 did not affect the viscosity of sols and improved their thermal stability. The electrochemical studies showed that the coatings were effective at protecting the metallic substrate. At a low concentration of cerium-modified clay particles (0.35 wt%), the La(III) played a role as an effective cathodic inhibitor of the
metallic substrate. In the end, it was concluded that the La(III) ions at the external layer of clay platelets induced the formation of La(OH)3, strengthening the corrosion protection of the OIH coating.
In considering lanthanum as a corrosion inhibitor, a study was undertaken on the electrochemical behavior of modified silane films with lanthanum triflate salt and/or silica NPs. The incorporation of LTS or silica NPs enhanced the anti-corrosion behavior of the silane films when compared to pure films. The films modified by both LTS and silica NPs showed improved corrosion
protection. These limitations were improved by incorporating La3+ cations in amorphous silica NPs before being embedded into the silane film. Furthermore, the coatings thus produced mitigated the corrosion process on copper bronze and lead, while steel substrates required thicker coatings.
Ideally, the addition of species to improve OIH coating performance must be incorporated within the coating matrix and released when required, i.e., when the corrosion process initiates, in order to delay further corrosion development. It has been reported in different studies that the direct incorporation of these species may interfere with the curing of the coating material and compromise its physico-chemical performance. Therefore, the selection of suitable carriers/nanocontainers, to ensure their release in response to a trigger, is of extreme importance. The trigger can be physical or chemical. Furthermore, these nanocontainers must be able to stock the required species in sufficient amounts and be compatible with the coating itself. The most commonly used reservoirs reported in the literature include urea-formaldehyde capsules, hydroxyapatite particles and halloysite nanotubes. Several OIH sol-gel coatings have been reported to have self-healing abilities.
The healing properties were confirmed through weight loss and electrochemical techniques. The same research group also studied the incorporation of the same corrosion inhibitors into aluminum pillared clay. The clay was dispersed as received within the matrix sol-gel coatings. The coatings mitigated the corrosion process during the beginning of exposure to an aggressive environment. Another coating in which the pillared clay was modified, by evacuation of a mixture of corrosion inhibitor, provided
improved corrosion protection for higher exposure periods in the same environment. This was shown by electrochemical and weight loss measurements. However, by increasing the coating exposure time in the aggressive environment, the coating suffered deterioration. It was also proven that the coatings provided autonomous healing in case of long-time exposure.
The self-healing mechanism in corrosion mitigation may be assessed and validated by several electrochemical tools. The most common techniques reported to assess the self-healing and corrosion inhibition of such materials are a scanning vibrating electrode technique (SVET), a scanning ion selective electrode technique (SIET) and localized impedance spectroscopy.
Sol-gel coatings with an anti-fouling function
Anti-fouling is another important function to enhance the corrosion protection of a coating. Coatings designed for this purpose are generally based on dense organic matrices containing a wide spectrum of biocides such as tributyltin and tributylin oxide.
These biocides are one of the major contaminants in marine and freshwater ecosystems, and nowadays their use is forbidden. Therefore, there is an emergent interest in developing new coatings with a controlled release of benign biocides. The use of nanocontainers loaded with biocides is considered to be a very attractive method, since a controlled release can minimize the environmental impact
Researchers have found that microbially influenced corrosion (MIC) and biofouling are prevented by the control of the microorganism activities in biofilms, their adhesion and their formation of biofilms. It was also concluded that the performance of antifouling coatings could be improved by the modification of self-polishing copolymers and the development of degradable polymers. Conductive polymers were also developed and introduced to mitigate corrosion and biofouling.
A wide range of encapsulated agents for fouling mitigation have been proposed and assessed, including enzymes, ZnO2, nanoparticles, silver and benzalkonium chloride, zinc pyrithione and chlorohexidine. The encapsulation of enzymes showed promise. The adsorption function of the peptide segments within peptide- polyethylene glycol (PEG) conjugates was weakened by enzymatic proteolysis. It was concluded that the adsorption properties related to the titanium substrate were fully suppressed,
showing potential for antifouling surfaces in the biomedical field. Coatings combining both anti-fouling and anticorrosion functions have been also reported.
It was concluded that to obtain a suitable balance between the hydrophobic properties and the film formation, the amount of polymer ranged between 75 and 85 wt%. The OIH coatings reported showed a water contact angle between 135º and 145º and good chemical resistance. The proposed system involved the use of a polyester-modified OIH-rich polysiloxane that was cured through reactions with different commercial isocyanate hardeners. The hydrophilic and hydrophobic domains were introduced within the system on the curing agent using polyethylene glycol (PEG)-ilate alkyl and cyclohexyl substituents, respectively, the prepared coatings from commercial precursors by dispersing a polydimethyl siloxane (silicone, PDMS), polyethylene glycol
triblock-copolymer in PDMS (PEG-PDMS-PEG).
Summary
The work on sol-gel technologies discussed here is only a small portion of the efforts currently underway. Developments are ongoing in several areas of study, including:
• Organic and inorganic hybrid materials
• Chemical and inorganic hybrid materials
• Chemical and biological properties
• Morphology and shape
• Biomedical applications
• Sol-gel bioactive glasses and hybrids
• Sol-gel based sensing devices
Sol-gel technology is being applied to hard anodizing and nanotechnology with nano-structures and pores. It is being integrated into applications in the pharmaceutical, biological, food industries, etc., with emphasis on surface treatments and choosing the right metal for specific uses. Sol-gel synthesis methods offer molecular-level mixing and are capable of improving the chemical homogeneity of the resulting composite.
Conclusions
Compatibility is but one facet of sol-gel derived biomedical applications. Sol-gel derived bioceramics have a great potential for application as a coating on metallic substrates to provide a high degree of biocompatibility and promote a rapid heating response with minimal averse biological events.
Since sol-gel processes are carried out at such low temperatures, they can also allow the inclusion of biomolecules, including therapeutic agents including drugs, growth factors and proteins. These can be loaded during the sol-gel preparation process and released in a controlled manner.
The sol-gel method should be simple stable, cost-effective and scalable for facilitating future industrial production and clinical translocation.
The corrosion mitigation/prevention of metallic substrates is not only an engineering effort, but also a fundamental scientific challenge. Ideally, an anticorrosion coating should be designed to be stimulus-responsive, multifunctional, resistant and durable in a wide range of applications.
The existing literature clearly shows that the distinctive properties of the OIH sol-gel materials can be successfully employed for the development of coating for corrosion mitigation of different metallic surfaces in challenging environments.
Although several OIH coatings for corrosion mitigation have been subjected to intensive research, further investigations are still necessary for both fundamental and applied aspects.
Bibliography
1. J.R. Jones, “Review of bioactive glass: From Hench to hybrids,” Acta Biomater., 9 (1), 4457-4486 (2013).
2. W.N.W. Ismail, “Sol-gel technology for innovate fabric finishing - A Review,” J. Sol-Gel Sci. & Technol., 78 (3), 698-707 (2016).
3. L. Rathinamala, N. Jeyakumaran and N. Prithivikumaran, “Sol-gel assisted spin coated CdS/PS electrode based glucose biosensor,” Vacuum, 161, 291-296 (2019).
4. J. Wu, et al., “Biomedical and clinical applications of immunoassays and immunosensors for tumor markers, Trends in Anal.Chem., 26 (7), 679-688 (2007).
5. S. Panneerselvam and S. Choi, “Nanoinformatics: Emerging Databases and Available Tools,” Int. J. Mol. Sci., 15 (5), 7158-7182 (2014).
6. R. Ciriminna, et al., “The sol-gel route to advanced silica-based materials and recent applications,” Chem. Rev., 113 (8), 6592-6620 (2013).
7. N. Le Bail, S. Benayoun and B. Toury, “Mechanical properties of sol-gel coatings on polycarbonate: A review,” J. Sol-gel Sci. & Technol., 75 (3), 710-719 (2015).
8. K. Lionti, et al., “Hybrid silica coatings on polycarbonate: Enhanced properties,” J. Sol-gel Sci. & Technol., 65 (1), 52-60 (2013).
9. E.K. Kim, et al., “Effects of silica nanoparticle and GPTMS addition on TEOS-based stone consolidants,” J. Cult. Heritage, 10 (2), 214-221 (2009).
10. W.J. Van Ooij and A. Sabata, “Characterization of films of organofunctional silanes by TOFSIMS and XPS,” J. Adhesion Sci. and Technol., 5 (10), 843-863 (1991).
11. A.R.B. Verma and W.J. van Ooij, “High-temperature batch hot dip galvanizing, Part 1: General description of coatings formed at 560°C,” Surf. and Coat. Technol., 89 (1-2),132-142 (1997).
12. L. FedrizziI, H. Terryn and A. Simoes, Eds., Innovative Pre-treatment Techniques to Prevent Corrosion of Metal Surfaces, Volume 54, 1st Edition, Elsevier, Amsterdam,The Netherlands, 2014; ISBN: 978-1-84569-368-8.
13. J.D. Wright and N.A.J.M. Sommerdijk, Sol-gel materials: Chemistry and Applications, 1st Edition, CRC Press, Boca Raton, FL, USA, 2000.
14. A. Balamurugan, S. Kannan and S. Rajeswari , “Structural and electrochemical behavior of sol-gel zirconia films on 316L stainless steel in simulated body fluid environment,” Mater. Lett., 57 (26-27), 4202-4205 (2003).
15. D.B. Haddow, P.F. James and R. VanNoort, “Sol-gel surfaces for biomedical applications,” J. Mater. Sci.: Mater. in Med., 7 (5), 255-260 (1996).
16. S. Radin and P. Ducheyne, “Controlled release of vancomycin from thin sol-gel films on titanium alloy fracture plate materials, Biomaterials, 28 (9), 1721-1729 (2007).
17. S.O. Pehkonen and S. Yuan, Tailored Thin Coatings for Corrosion Inhibition Using a Molecular Approach; Academic Press, Cambridge, MA, USA, 2018; ISBN 978-0-12-813584-6.
18. J. Bhandari, et al., “Modelling of pitting corrosion in marine and offshore steel structures: A technical review”, J. Loss Prev. in the Process. Ind., 37, 39-62 (2015).
19. The Global Cost and Impact of Corrosion, NACE International https://inspectioneering.com/news/2016-03-08/5202/nacestudy-estimates-global-cost-of-corrosion-at-25-trillion-ann (last accessed on 3 September 2021).
20. Market Research Reports, Industry Analysis, Business Overview & Trends: 360 Market Updates, https://www.360marketupdates.com/ (last accessed on 3 September 2021).
21. R.V. Lakshmi, S.T. Aruna and S. Sampath, “Ceria nanoparticles vis-à-vis cerium nitrate as corrosion inhibitors for silicaalumina hybrid sol-gel coating,” Appl. Surf. Sci., 393, 397-404 (2017).
22. D-M. Liu,et al., “Structural evolution of sol-gel-derived hydroxyapatite,” Biomaterials, 23 (7),1679-1687 (2002).
23. F. Foroutan, et al., “Novel sol-gel preparation of (P2O5)0.4–(CaO)0.25–(Na2O)X–(TiO2)(0.35−X) bioresorbable glasses (X = 0.05, 0.1, and 0.15),” J. Sol-gel Sci. and Technol., 73 (2), 434-442 (2014).
24. R. Ciriminna, et al., “From molecules to systems; sol-gel microencapsulation in silica-based materials,” Chem. Rev., 111 (2), 765-789 (2011).
25. W. Stober, A. Fink and E. Bohn, “Controlled growth of monodisperse silica spheres in the micron size range,” Colloid Interface Sci., 26 (1), 62-69 (1968).
26. R. Barbey, et al., “Polymer brushes via surface-initiated controlled radical polymerization: synthesis, characterization, properties, and applications,” Chem. Rev., 109 (11), 5437-5527 (2009).
27. M.A.C. Stuart, et al., “Emerging applications of stimuli-responsive polymer materials,” Nature Mater., 9 (2), 101-113 (2010).
28. J.D. Berrigan, et al., “Protein-enabled layer-by-layer syntheses of aligned, porous-wall, high-aspect-ratio TiO2 nanotube arrays,” Adv. Funct. Mater., 21 (9), 1693-1700 (2011).
29 P.C.R. Varma, et al., “Corrosion protection of AA 2024-T3 aluminum alloys using 3,4 diaminobenzoic acid chelated zirconium-silane hybrid sol-gels,” Thin Solid Films, 518 (20), 5753-5761 (2010).
30 M. Catauro, et al., “Corrosion behaviour and mechanical properties of bioactive sol-gel coatings on titanium implants,” Mater. Sci. Eng C: Mater. Bio. Appl., 43, 375-382 (2014).
About the author
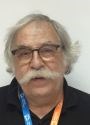
Dr. Xavier Albort Ventura earned his Ph.D. in Chemical Engineering Industrial from the University of Barcelona Spain. He is a member of the Barcelona International Trade Fair and has organizing responsibility for the Environment Technical Meetings and at the Eurosurfas show. He is an electroplating consultant for more than 50 European companies from various countries, including Portugal, France, Spain, Italy and Germany. Dr. Ventura has been an active presenter at SUR/FIN, having given papers since
1987. He has also been an active participant at Interfinish, presenting papers and conducting workshops since 1984. He has published more than 200 papers in Spain, the United Kingdom, France, the United States and Brazil, and has won a number of national and international awards in the United States. Collaboration in technical books with written chapters on in compilation of different conference authors in a dozen books. He received the Sam Wyman Memorial Award at SUR/FIN 2004 in Chicago for the Best Light Metals paper, “Sealing Method for Anodizing Aluminum and Hard Anodizing and a Test to Determine the Seal Quality of Aluminum.”
Related Content
Calculating Applied Media Force During Vibratory Finishing
What appear to be identically set-up vibratory bowls will finish identical loads of parts in varying time cycles. This paper offers a new technique to better predict what the operator will produce, by measuring the force applied to the parts. It is the efficiency of that force which controls the efficiency and speed of the refinement cycle.
Read MoreSUR/FIN 2023: Capsules from the Technical Sessions I: Emerging Technologies
SUR/FIN 2023 in Cleveland this past June was a resounding success. Due to the efforts of the Technical Activities Committee, ably led by Bill Nebiolo this year, an outstanding program of technical presentations was offered. What follows are summaries of selected presentations from the Emerging Technologies sessions. Additional coverage will be provided in this space in the coming months. The full report can be accessed and printed at short.pfonline.com/NASF23Aug1.
Read MoreHexavalent-Chromium-Free Aluminum Sacrificial Paint Validation
Hexavalent chromium is a known carcinogen, repro-toxin and mutagen. Its elimination is of high importance to the aerospace industry, which has struggled to find high performing alternatives. Legacy aluminum sacrificial paints have traditionally utilized hexavalent chromium to prevent corrosion and coatings which are equal to or better than have been difficult. This second of two papers discusses the hexavalent-chromium-free process from the user point-of-view in terms of the process validation work by Rolls Royce Corporation.
Read MoreNASF's SUR/FIN 2023: Bringing the Surface Finishing Industry Together
SUR/FIN 2023 is an opportunity for those in the surface finishing industry to expand their knowledge, expertise and network.
Read MoreRead Next
Episode 42: An Interview with Robin Deal, Hubbard-Hall
Hubbard-Hall wastewater treatment specialist Robin Deal discusses the latest trends in wastewater management.
Read MorePowder Coating 4.0: Smarter, Faster, More Efficient and Connected
New tools reduce cost and waste, lower manufacturing footprint of powder coating operations.
Read MoreThe 2024 Ford Mustang: All the Colors Available
Although Chevrolet has announced the end of the Camaro and Dodge is offering “Last Call” editions of the Charger and Challenger, the Ford Mustang is launching to its seventh generation.
Read More