NASF/AESF Foundation Research Project #123: Electrochemical Manufacturing for Energy Applications - 1st Quarterly Report
For 2022, NASF-AESF Foundation Research Board has selected a project on electrodeposition toward developing low-cost and scalable manufacturing processes for hydrogen fuel cells and electrolysis cells for clean transportation and distributed power applications. This report introduces the project and outlines the work to be undertaken.
by
Majid Minary Jolandan
Associate Professor of Mechanical and Aerospace Engineering
School for Engineering of Matter, Transport and Energy
Ira A. Fulton School of Engineering
Arizona State University
Tempe, Arizona, USA
Editor’s Note: For 2022, NASF-AESF Foundation Research Board has selected a project on electrodeposition toward developing low-cost and scalable manufacturing processes for hydrogen fuel cells and electrolysis cells for clean transportation and distributed power applications. This report introduces the project and outlines the work to be undertaken. A printable PDF version of this report is available by clicking HERE.
Introduction and review of literature
Solid oxide fuel cells (SOFCs) are electrochemical devices that generate electricity by direct conversion of chemical energy of fuels (H2, CO, CH4, etc.) through electrochemical reactions. They have enormous potential for commercial applications given their high efficiency, high energy density and fuel flexibility.1–4 In general, SOFCs consist of three major components, namely two porous composite electrodes, referred to as the fuel electrode (anode) and air electrode (cathode), and a dense electrolyte layer.5 SOFCs are powered by redox reactions of oxygen and fuel at the three-phase boundary (TPB), where the gas phase, electron-conducting phase, and ionic-conducting phase collide to form the electrochemical reaction.6,7 The fuel is oxidized at the fuel electrode (anode) side. The anode electrode assists the fuel adsorption and catalyzes the oxidation reactions by the combination with oxide ions to bring forth the electron for the electric current. The reduction of oxygen produces oxygen ions at the cathode site. These oxygen ions diffuse through the dense layer of solid electrolyte to the anode. The electrons are then liberated to the outer circuit, i.e., the cathode side, to complete the energy generation circle. It is therefore critical to have porous structures for the enhancement of catalytic activity in the anode electrode.8,9
To serve this purpose, the nickel-yttria-stabilized zirconia (Ni-YSZ) has been found to be the most effective anode electrode for intermediate-temperature SOFCs that operate below 800°C.10 Nickel is stable under reducing conditions and able to react as a catalyst to promote the fuel oxidation process. Furthermore, in amalgamation with the porous YSZ structure, Ni facilitates the conduction of oxide ions in the electrode component. Thus, Ni-YSZ is preferred for use in single cell SOFC construction. Therefore, it is critical to choose the appropriate manufacturing method for producing the porous anode electrode.11,12 On the other hand, the cathode occupies the other side of the anode while sandwiching the electrolyte, controls the whole cell that influences the performance of the output system.
The performance of the cathode however, can be degraded by poisoning and corrosion issues caused by external contaminants, such as oxidants from the surrounding air which contains pollutants. For example, long-term exposure to carbon dioxide and humidity in the air during the operating cycle may lead to corrosion of the cathode.13 The most frequent type of material used in SOFC applications is perovskite (calcium titanate, CaTiO3), while the most common electrode is strontium-doped lanthanum manganite (LSM). The high conductivity of LSM-based electrodes up to 100 S/cm at 600°C and the well-matched coefficient of thermal expansion with the YSZ electrolyte are two benefits. To avoid the degradation of the cell, it is also suggested that the components of the fuel cycle should be compatible, so that thermo-mechanical stress is minimized for optimal SOFC performance.14 Therefore, it is critical to select the appropriate fabrication method for integrating electrolyte and cathode electrode elements.
The electrolyte should be adequately densified to prevent fuel/oxidant gas leakage to the electrodes and to lower the resistance to oxygen ion transport in the electrolyte. To prevent losses due to leakage current, it is expected that the electronic conductivity of the electrolyte to be lower as well. Flaws, pinholes and other defects are the potential reasons for the drastic reduction of the electrochemical performance of the fuel cell. To reduce the manufacturing defects and ensure high density, the sintering step of the electrolyte is, therefore, vital. The YSZ electrolyte can be sintered in the temperature range of 1300-1500°C.15 The density of the electrolyte is a critical factor in maximizing the electrical conductivity of the electrolyte. The nominal electrical conductivity of the SOFC electrolyte should be in the range of 2-4 × 10-2 S/cm for practical applications.16 Because of the compatibility and low chemical reactivity with YSZ, as well as their similar coefficients of thermal expansion, LSM is more commonly used for the oxygen electrode.
To date, a variety of manufacturing techniques such as extrusion,17-19 isostatic pressing,17,20 tape casting21,22 and slip casting23,24 have been adopted to fabricate porous anode material. In these conventional processes, the pores are generated by mixing the anode powder with pore-formers and burning out the pore-formers during the sintering process. These pores are generally distributed in a random manner in the anode substrate,25,26 resulting in a significant number of isolated pores not being interconnected. This limits the gas diffusion in Ni-YSZ supports of SOFCs.27-29 Additionally, this random distribution of pores leads to higher tortuosity of the anode, which is not a favorable condition for the gas diffusion in the electrodes.30
To overcome this problem, a freeze-casting technique can be beneficial to create a functionally-graded support with pore channels formed by the ice crystal growth.31,32 Freeze-cast structures exhibit several advantages, including low tortuosity and high porosity that enables rapid gas diffusion. Apart from these properties, freeze-cast electrodes and supports offer low polarization resistance, high electrochemical performance and facilitate catalyst infiltration, as they have straight pore channels.33 Wei, et al.34 reported the fabrication of metal supports for SOFC with vertically aligned porous structures using the freeze-casting technique. The freeze-casted planar single cells developed by the NASA Glenn Research Center using the freeze-casting method have exhibited a significantly high specific power density of 1.0 kW/kg due to the low tortuosity of acicular pores in the anode support.35 Frequently, several planar freeze-cast fabricated fuel cells have been reported in recent years.34-38 However, low thermocyclic durability and slow startup times are several common issues for planar solid oxide fuel cells.
In our research, a directional freeze-casting method will be implemented to prepare porous anode scaffolds of YSZ, and Ni will be deposited inside the open pore structures by electrodeposition. Traditionally, Ni-YSZ anodes are fabricated by a powder metallurgy process through mechanical mixing of NiO and YSZ, which is subsequently followed by sintering at temperatures from 1300 to 1500°C and reduction in a hydrogen-rich atmosphere. This method requires a large amount of Ni (greater than 30 vol%) to achieve sufficient electronic conductivity. Additionally, the process involves high temperature reduction in hydrogen, which itself an energy intensive process, and thus, increases the cost of fabrication. Therefore, the proposed method of fabricating Ni-YSZ anode cermets will not only be cost effective but also will be energy efficient. Electrodeposition of Ni into the porous YSZ scaffolds might also be effective to enhance the electrochemical activity of the electrodes through the deposition of catalytically active nanoparticles and minimizing the cell’s ohmic resistance through the formation of a thin-film electrolyte. This technique can also hinder the current leakage or chemical interaction between functional layers by depositing thin buffer layers.39 Additionally, infiltration by electrodeposition will allow a wider range of composition, in particular for metals that cannot be processed at high temperatures such as copper.
Framework for Fabrication of Ni-YSZ Anode Cermet
The proposed framework for the fabrication of Ni-YSZ cermet is shown in Fig. 1 below.
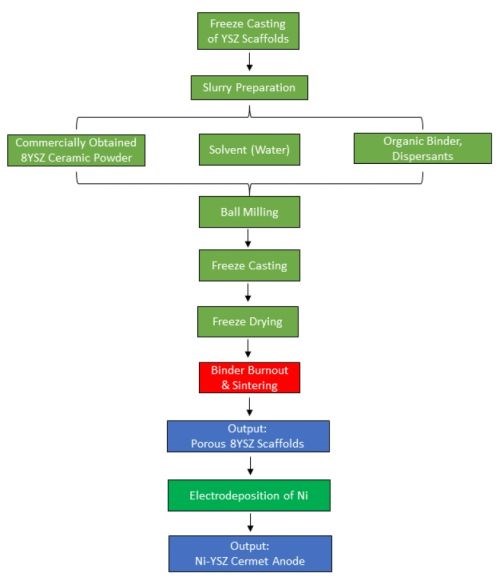
Figure 1 - Framework for the fabrication of Ni-YSZ SOFCs anode electrode
References
1. R. O’Hayre, S-W. Cha, W. Colella and F.B. Prinz, Fuel Cell Fundamentals, 3rd Edition, John Wiley & Sons, 2016.
2. R.Ø. Gadsbøll, J. Thomsen, C. Bang-Møller, J. Ahrenfeldt, and U.B. Henriksen, “Solid oxide fuel cells powered by biomass gasification for high efficiency power generation,” Energy, 131, 198-206 (2017).
3. K. Zhao and Y. Du, “Calcium-doped ceria materials for anode of solid oxide fuel cells running on methane fuel,” Journal of Power Sources, 347, 79-85 (2017).
4. S. Farhad, F. Hamdullahpur and Y. Yoo,” Performance evaluation of different configurations of biogas-fuelled SOFC micro-CHP systems for residential applications,” International Journal of Hydrogen Energy, 35 (8), 3758-3768 (2010).
5. J. Verma, M. Vijayakumar and R. Mitra, “Processing and microstructure of freeze-cast silica foams,” Materials Letters, 153, 168-170 (2015).
6. J.J. Spivey and S.S. Chuang, Catalysis of solid oxide fuel cells, RSC Publications, The Royal Society of Chemistry, Cambridge, UK, 2005.
7. N. Hedayat, Y. Du and H. Ilkhani, “Review on fabrication techniques for porous electrodes of solid oxide fuel cells by sacrificial template methods,“ Renewable and Sustainable Energy Reviews, 77, 1221-1239 (2017).
8. L. Bi, E.H. Da’as and S.P. Shafi, “Proton-conducting solid oxide fuel cell (SOFC) with Y-doped BaZrO3 electrolyte,” Electrochemistry Communications, 80, 20-23 (2017).
9. M.S. Khan, S-B. Lee, R-H. Song, J-W. Lee, T-H. Lim andf S-J. Park, “Fundamental mechanisms involved in the degradation of nickel–yttria stabilized zirconia (Ni–YSZ) anode during solid oxide fuel cells operation: A review,” Ceramics International, 42 (1), 35-48 (2016).
10. Z. Zakaria, Z. Awang Mat, S.H. Abu Hassan and Y. Boon Kar, “A review of solid oxide fuel cell component fabrication methods toward lowering temperature,” International Journal of Energy Research, 44 (2), 594-611 (2019).
11. D. Kennouche, Y-C.K. Chen-Wiegart, C. Riscoe, J. Wang and S.A. Barnett, “Combined electrochemical and X-ray tomography study of the high temperature evolution of Nickel–Yttria Stabilized Zirconia solid oxide fuel cell anodes,” Journal of Power Sources, 307, 604-612 (2016).
12. D. Kennouche, Y-C.K. Chen-Wiegart, K.J. Yakal-Kremski, J. Wang, J.W. Gibbs, P.W. Voorhees and S,A, Barnett, “Observing the microstructural evolution of Ni-Yttria-stabilized zirconia solid oxide fuel cell anodes,” Acta Materialia, 103, 204-210 (2016).
13. Z. Yang, M. Guo, N. Wang, C. Ma, J. Wang and M. Han, “A short review of cathode poisoning and corrosion in solid oxide fuel cell,” International Journal of Hydrogen Energy, 42 (39), 24948-24959 (2017).
14. Z. Gao, L.V. Mogni, E.C. Miller, J.G. Railsback and S.A. Barnett,” A perspective on low-temperature solid oxide fuel cells,” Energy & Environmental Science, 9 (5), 1602-1644 (2016).
15. B. Xing, C. Cao, W. Zhao, M. Shen, C. Wang and Z. Zhao, “Dense 8 mol% yttria-stabilized zirconia electrolyte by DLP stereolithography,” Journal of the European Ceramic Society, 40 (4), 1418-1423 (2020).
16. J.W. Fergus, “Electrolytes for solid oxide fuel cells,” Journal of Power Sources, 162 (1),30-40 (2006).
17. Y. Du and N.M. Sammes, “Fabrication and properties of anode-supported tubular solid oxide fuel cells,” Journal of Power Sources, 136 (1), 66-71 (2004).
18. W-S. Hsieh, P. Lin and S-F. Wang, “Fabrication of electrolyte supported micro-tubular SOFCs using extrusion and dip-coating,” International Journal of Hydrogen Energy, 38 (6), 2859-2867 (2013).
19. J-H. Song and N.M. Sammes, “Fabrication of anode-supported tubular solid oxide fuel cell using an extrusion and vacuum infiltration techniques,“ Journal of Fuel Cell Science and Technology, 7 (6), 61013 (2010).
20. K. Zhao, B-H. Kim, Q. Xu and B-G. Ahn, “Fabrication and characterization of inert-substrate-supported tubular single cells by dip-coating process,” Journal of Power Sources, 245, 671-677 (2014).
21. A. Modjtahedi, N. Hedayat and S.S.C. Chuang, “The direct carbon solid oxide fuel cell with H2 and H2O feeds,” Solid State Ionics, 268, 15-22 (2014).
22. L. Zhang, M. Li, X. Song, T. Guo, S. Zhu, W. Ji and H. Wang, “Preparation of half-cell by bi-layer wet powder spraying and tape casting for anode-supported SOFCs.” Journal of Alloys and Compounds, 586, 10-15 (2014).
23. Y. Zhang, J. Liu and J. Yin, “ Fabrication of anode-supported tubular solid oxide fuel cells by slip casting in combination with dip coating technique,” Journal of Fuel Cell Science and Technology, 7 (1), 011014 (5 pp.)(2010).
24. L. Zhang, H.Q. He, W.R. Kwek, J. Ma, E.H. Tang and S.P. Jiang, “Fabrication and characterization of anode‐supported tubular solid‐oxide fuel cells by slip casting and dip coating techniques,” Journal of the American Ceramic Society,” 92 (2), 302-310 (2009).
25. W. Schafbauer, N.H. Menzler and H.P. Buchkremer, “Tape casting of anode supports for solid oxide fuel cells at Forschungszentrum Jülich,” International Journal of Applied Ceramic Technology, 11 (1), 125-135 (2012).
26. M. Letilly, O. Joubert, M-T. Caldes and A.L.G. La Salle, “Tape casting fabrication, co-sintering and optimisation of anode/electrolyte assemblies for SOFC based on BIT07-Ni/BIT07.” International Journal of Hydrogen Energy, 37 (5), 4346-4355 (2012).
27. A. Modjtahedi, N. Hedayat and S.S.C. Chuang, “Diffusion-limited electrochemical oxidation of H2/CO on Ni-anode catalyst in a CH4/CO2-solid oxide fuel cell,” Catalysis Today, 278 (2), 227-236 (2016).
28. O.A. Marina and M. Mogensen, “High-temperature conversion of methane on a composite gadolinia-doped ceria–gold electrode,” Applied Catalysis A: General, 189 (1), 117-126 (1999).
29. K.J. Yoon, S-I. Lee, H. An, J. Kim, J-W. Son, J-H. Lee H-J. Je, H-W. Lee and B-K. Kim, “Gas transport in hydrogen electrode of solid oxide regenerative fuel cells for power generation and hydrogen production,” International Journal of Hydrogen Energy, 39 (8), 3868-3878 (2014).
30. W. Kong, Q. Zhang, X. Gao, J. Zhang, D. Chen and S. Su, “A method for predicting the tortuosity of pore phase in solid oxide fuel cells electrode,” International Journal of Electrochemical Science, 10 (7), 5800-5811 (2015).
31. C. Gaudillere and J.M. Serra, “Freeze-casting: Fabrication of highly porous and hierarchical ceramic supports for energy applications,” Boletín de la Sociedad Española de cerámica y vidrio, 55 (2), 45-54 (2016).
32. R. Liu, T. Xu and C-A. Wang, “A review of fabrication strategies and applications of porous ceramics prepared by freeze-casting method,” Ceramics International, 42 (2) Part B, 2907-2925 (2016).
33. Y. Du, N. Hedayat, D. Panthi, H. Ilkhani, B.J. Emley and T. Woodson, “Freeze-casting for the fabrication of solid oxide fuel cells: A review,” Materialia, 1, 198-210 (2018).
34. P. Wei, S. Sofie, Q. Zhang and A. Petric, “Metal supported solid oxide fuel cell by freeze tape casting,” ECS Transactions, 35 (1), 379-383 (2011).
35. T.L. Cable and S.W. Sofie, “A symmetrical, planar SOFC design for NASA’s high specific power density requirements,” Journal of Power Sources, 174 (1), 221-227 (2007).
36. M.M. Porter, L. Meraz, A. Calderon, H. Choi, A. Chouhan, L. Wang, M.A. Meyers and J. McKittrick, “Torsional properties of helix-reinforced composites fabricated by magnetic freeze casting,” Composite Structures, 119, 174-184 (2015).
37. A.Z. Lichtner, D. Jauffrès, D. Roussel, F. Charlot, C.L. Martin and R.K. Bordia, “Dispersion, connectivity and tortuosity of hierarchical porosity composite SOFC cathodes prepared by freeze-casting,” Journal of the European Ceramic Society, 35 (2), 585-595 (2015).
38. P.M. Hunger, A.E. Donius and U.G.K. Wegst, “Structure-property-processing correlations in freeze-cast composite scaffolds,” Acta Biomaterialia, 9 (5), 6338-6348 (2013).
39. E. Kalinina and E. Pikalova, “Opportunities, challenges and prospects for electrodeposition of thin-film functional layers in solid oxide fuel cell technology,” Materials, 14 (19), 5584 (2021).
About the author

Majid Minary Jolandan is Associate Professor, Mechanical and Aerospace Engineering, School for Engineering of Matter, Transport and Energy, in the Ira A. Fulton School of Engineering, at Arizona State University, Tempe, Arizona. His group is interested in various manufacturing processes to address current national challenges in advanced materials. Current interests in his group include advanced and additive manufacturing and multifunctional and smart materials.
His education includes B.S. Sharif University of Technology (2003), M.S. University of Virginia (2005), Ph.D. University of Illinois at Urbana-Champaign (2010) as well as Postdoctoral fellow, Northwestern University (2010-2012). From 2012-2021, he held various academic positions at The University of Texas at Dallas and joined the Faculty at Arizona State in August 2021.
He teaches advanced manufacturing, mechanical behavior of materials and structural mechanics in the School for Engineering of Matter, Transport and Energy.
Early in his career, he received the Young Investigator Research Program grant from the Air Force Office of Scientific Research to design high-performance materials inspired by bone that can reinforce itself under high stress. This critical research can be used for aircraft and other defense applications, but also elucidate the understanding of bone diseases like osteoporosis.
He also earned the Junior Faculty Research Award as an assistant professor at the University of Texas-Dallas School of Engineering.
Related Content
How to Choose Between Sulfate and Chloride-Based Trivalent Chromium
There are several factors to consider when choosing between sulfate and chloride-based baths for trivalent chromium plating. Mark Schario of Columbia Chemical discusses the differences and what platers should keep in mind when evaluating options.
Read MoreInnovation in Plating on Plastic
Plating on advanced plastics solution offers improved adhesion, temperature resistance and cost savings.
Read More3 Tests to Ensure Parts are Clean Prior to Plating
Making sure that all of the pre-processing fluids are removed prior to plating is not as simple as it seems. Rich Held of Haviland Products outlines three tests that can help verify that your parts are clean.
Read MoreHow to Maximize Nickel Plating Performance
The advantages of boric acid-free nickel plating include allowing manufacturers who utilize nickel plating to keep up the ever-changing regulatory policies and support sustainability efforts.
Read MoreRead Next
Education Bringing Cleaning to Machining
Debuting new speakers and cleaning technology content during this half-day workshop co-located with IMTS 2024.
Read MoreDelivering Increased Benefits to Greenhouse Films
Baystar's Borstar technology is helping customers deliver better, more reliable production methods to greenhouse agriculture.
Read MoreEpisode 45: An Interview with Chandler Mancuso, MacDermid Envio Solutions
Chandler Mancuso, technical director with MacDermid Envio discusses updating your wastewater treatment system and implementing materials recycling solutions to increase efficiencies, control costs and reduce environmental impact.
Read More