Choosing and Troubleshooting Copper Electroplating Processes
Learn more on this inexpensive and highly efficient process.
Share
Read Next
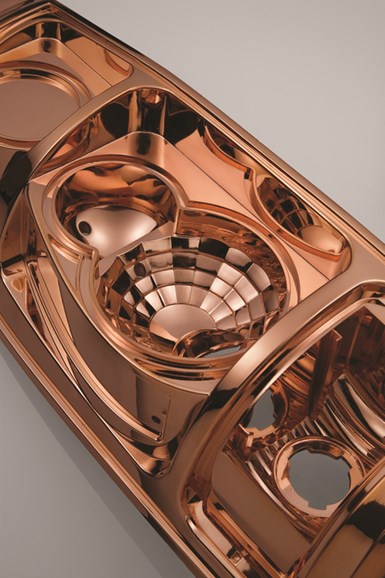
Excluding the specialty and continuous-strip plating industries, more copper is plated than any other decorative metal except for nickel. There are several reasons for this:
- Copper is an excellent undercoat for subsequent deposits, since it's a good metal to cover substrate defects that can accelerate corrosion. Buffing copper even improves its ability to be an excellent substrate. Copper, and especially buffed copper, can provide an easy-to-plate, highly level and bright substrate.
- Copper is among the least expensive metals and is in relatively stable supply. More leveling can be obtained for the cost of the metal electroplated than for any other metal.
- Copper has a high plating efficiency, and many copper plating processes offer good coverage and throwing power.
- Copper metal is less environmentally hazardous than many other plated metals, although the EPA limits the discharge of copper and copper plating solutions. The waste treatment processes for copper plating solutions and copper-containing rinse waters are well known and in most cases easy to accomplish. Also, many times the copper metal and even the plating bath can be recycled.
- Copper’s high electrical conductivity is exceeded only by silver, making it an excellent and inexpensive coating for products such as printed circuit boards. Used as a topcoat on steel wire, it produces high-strength, conductive electrical cable.
- The percent elongation of most electroplated copper deposits is greater than other electroplated metals. This property helps substrates such as plastic and aluminum withstand thermal expansion without cracking their electrodeposited coatings.
Three basic types of processes are available based upon the complexing system used: alkaline (several modifications of cyanide and non-cyanide); acid (sulfate and fluoborate) and mildly alkaline (pyrophosphate) complexed baths.
Alkaline Cyanide Solutions
Alkaline copper solutions have better throwing power (uniform deposit thickness) than acid copper solutions. However, they cannot be used at as high a current density as acid copper. They are also relatively more difficult to control than acid sulfate solutions. Also, cyanide involves special handling and treatment procedures because of its acute health hazards and waste disposal requirements. But, the procedures are well understood, and, with care, cyanide can be handled safely.
Types of cyanide copper baths. A cyanide copper solution can be used in either rack or barrel plating. The processes can be generally classified as low-efficiency or “strike” processes, medium-efficiency “Rochelle” processes and high-metal, high-efficiency or “high-speed” processes.
A cyanide copper strike bath is typically used to deposit a thin, adherent layer that can completely cover an active metal surface such as zinc or steel prior to further plating operations. Because of the bath’s low plating efficiency, plating time, and thus the deposit’s thickness, is often determined by the time needed to just obtain complete coverage.
The maximum practical deposit thickness is typically in the range of 1.2–2.5 µm (0.05–0.10 mil). The copper strike serves only as a protective layer for further plating, typically with copper or nickel. It also improves adhesion and, in some cases, acts as “insurance” in the pre-plate cycle to obtain adhesion because cyanide can function as a cleaner/activator.
The low-metal and high-cyanide levels in the copper strike are responsible for the low efficiency, but these same properties ensure against a non-adhering immersion layer of copper forming on the surface being plated. This formulation also produces the desired excellent covering and throwing powers.
The Rochelle salt bath is often used for similar purposes. But, it may also be used to provide thicker deposits than can be obtained with cyanide strike baths.
The high-efficiency formulation, with its higher temperature and copper concentration, plates faster and can produce thicker deposits. However, it has lower covering power. Therefore, a cyanide strike must be used prior to this bath when parts with low-current-density areas are plated. The trend is to use cyanide copper baths just for strikes and use other processes such as acid sulfate copper to increase deposit thickness.
Carbonate Chemistry. Carbonate, typically as sodium carbonate, is added to strike and Rochelle salt processes at bath makeup. It controls pH by acting as a buffer and reduces anode polarization.
Carbonate is not added to high-efficiency baths. However, carbonate forms naturally during operation in all cyanide baths. This is a result of the decomposition of free cyanide through hydrolysis and cyanide oxidation at the anode. Carbonate also builds up in the bath by absorption of carbon dioxide from the atmosphere, an effect promoted by air agitation.
If carbonate concentration reaches 75–100 g/L, some should be removed since it reduces the bright plating range. Remove carbonates by precipitation through the addition of calcium (lime) or barium hydroxide to form insoluble calcium or barium salts. Barium cyanide, which also lowers carbonate content, prevents an increase in the hydroxide level of the bath.
Another approach is to chill the sodium-based bath to form sodium carbonate, which is much less soluble at lower temperatures. If the temperature is reduced below 32°F, copper salts also will precipitate. Carbonates cannot be chilled out of a potassium-based bath, since potassium carbonate is too soluble.
For both the precipitation and chilling procedures, use a treatment tank other than the plating tank. This allows the carbonate to settle out before pumping the plating solution through a filter into a cleaned plating tank.
Anodes, current. Use high-purity, oxide-free anodes for high-efficiency decorative copper plating. Lower quality anodes can be used in strike and medium-efficiency Rochelle-salt cyanide-copper plating baths (provided that anode current density is kept low). Copper balls or nuggets in steel baskets, or slab anodes may be used. Intermingle plain steel anodes with the copper anodes to control the buildup of copper in the bath. Keep the anode/cathode ratio between 1:1 and 2:1. It’s also a good idea to bag the anodes (PP, nylon) in order to contain insoluble particles coming from the anodes.
Current interruption and periodic reverse (PR) are beneficial in high-efficiency processes, since they help provide brighter and smoother deposits. Current interruption cycles are typically 10 sec on and one sec off. Typical PR cycles require 10–60 sec direct current followed by 2–20 sec of reverse current. Processes using additives are available that do not require current interruption to produce bright deposits.
Troubleshooting, purification. The best method is to prevent the problem in the first place. Chemical analysis and frequent Hull cell testing will avoid many common problems. Eliminate the source of the problem so that purification or other corrective measures will not need to be conducted as often.
All cyanide copper plating baths, especially the high-efficiency baths, are sensitive to contaminants. Organic residues from buffing compounds, cleaners, tank and rack materials and other organics also can contaminate the bath.
Continuously filtering the plating solution through a filter chamber packed with approximately 0.7 g of activated plating-grade carbon per liter of plating solution (0.1 oz/gal) will remove small amounts of some organics. Change the carbon frequently and properly dispose of the carbon. Once the carbon has been in contact with the cyanide copper electrolyte, it is contaminated with organics and cyanide and must be treated as hazardous.
If a carbon-packed filter is not available or if the organic contamination is too severe, a batch carbon/peroxide treatment may be necessary. Since this is best accomplished in a tank other than the plating tank, chilling the solution to remove carbonates may be done at the same time. Table II gives directions for a typical batch carbon/peroxide treatment.
Even low concentrations of hexavalent chromium, which can be dragged into the bath on the racks, will produce dull and spotty deposits. Chromium can be reduced quickly to its trivalent form and precipitated by the addition of proprietary reducing agents directly to the plating bath. Hexavalent chromium also can be converted to the inactive trivalent state by means of high-current-density electrolysis or by adding sodium hydrosulfite or sodium stannite. There is disputed evidence that trivalent chromium left in the plating bath can be reoxidized by air back to hexavalent chromium. The best procedure is to precipitate the chromium and remove it by filtering.
Zinc contaminants will plate out simultaneously with the copper, causing brittle and brassy looking deposits. Zinc can be removed by dummying the bath at 0.2–0.4 A/dm2. Dummying also removes many other metallic contaminants.
Sulfur and its compounds cause dull, red deposits in low-current-density areas. These usually appear in deposits from new baths as a result of the use of impure cyanides or leaching out contaminants from tank linings, racks and anode bags.
Alkaline, Non-Cyanide Solutions
Concern for worker safety and government regulations on cyanide have made the use of non-cyanide coppers desirable. However, it is questionable if they can replace cyanide processes in all applications, especially for rack plating zinc die castings, due to the formation of a non-adhering immersion copper deposit. A process should only be used after extensive testing on the actual parts to be plated, produced under production conditions. The users should also confirm that the proprietary non-cyanide complexer used to replace cyanide is less hazardous and easier to waste treat than cyanide.
Many non-cyanide copper processes operate between 54–65°C (130–150°F) with air agitation. A typical bath formulation contains 11g/L of copper metal. The pH is around 9.0, while cyanide copper solutions have a pH of at least 11.0. The anode-to-cathode ratio is 2:1, with the same anodes used in cyanide copper solutions.
Analysis and routine additions of the complexer are all that are typically needed to maintain the bath. However, the removal of metallic impurities might be a major problem with some processes.
Acid Copper Solutions
Acid copper baths are simple formulations, containing copper ions, additives and either sulfate or fluoborate ions along with the corresponding acids. Because of their acidity, they cannot be plated directly onto active metals, such as zinc die castings and steel, for they will produce non-adhering immersion deposits.
The chemical cost of acid copper baths is low, and they can have a wide range in composition. When compared with cyanide and alkaline non-cyanide baths, their effluent control is simpler, they are easier to control and they are more stable. Their anode and cathode efficiencies are high, close to or equal to 100%. With high agitation they can tolerate high current densities.
However, because of their low cathode polarization, the acid baths do not have throwing power as good as that of alkaline solutions, making them poor strike baths.
Chemistry
Table III shows the chemical makeup and operating conditions of typical acid copper sulfate baths. General purpose baths are used for decorative plating, while high-throw and high-speed baths are for special applications such as printed circuit board and strip plating. High-throw baths are formulated to plate more copper in the very low current density holes and less on the surface of circuit boards than other acid copper processes. A high-speed bath can plate about twice as fast as conventional baths while retaining all the desired deposit properties.
The concentration of copper sulfate helps determine the properties of the baths. At higher concentrations, the resistivity of the bath is greater, and the anode and cathode polarization are slightly reduced. At lower copper sulfate concentrations, throwing power increases. A concentration of less than 60 g/L of copper sulfate decreases cathode efficiency. The solubility of copper sulfate decreases with increasing sulfuric acid concentration.
Sulfuric acid gives the bath its high conductivity, reduces anode and cathode polarization and prevents precipitation of basic copper salts. A practical minimum sulfuric acid concentration is about 45 g/L.
Agitation, anodes. To ensure brightness and to prevent high-current-density burning, agitation of acid copper baths is essential. Air agitation from an oil-free blower is best for decorative copper plating. For PC boards, mechanical agitation, in which the PC board’s movement forces solution through the board’s holes, is good to obtain maximum throwing power. For high-speed copper plating at high current density, high-velocity solution flow and/or part movement, perpendicular to the cathode, has been successful.
A necessary black cupric oxide film forms on the anodes. If it is disturbed, such as with excessive high anode current density, brightener consumption and roughness will increase, deposit ductility will decrease and leveling of deposits will be reduced. If the anodes have either a pink or a light gray appearance, too low anode phosphorus content or electrical problems may be the cause. Low-current-density electrolysis, starting at 0.5 and building up to 2.5 A/dm2 (5–25 asf), should develop the desired film if the correct anode material is used.
Contamination. Nickel, cobalt, chromium and iron will not readily co-deposit with copper but will reduce the solution’s conductivity when a total of about 1,000 ppm are present. These metals cannot be removed.
Iron will also cause the copper concentration in the bath to increase by a reaction with the anode during idle periods.
Calcium and lead will precipitate as sulfates and cause roughness if not removed, but they do not affect the deposit.
Tin can co-deposit to cause rough dark deposits if present above 60 ppm. Lead and tin are usually introduced into the bath by carry-over on solder plated racks. Stripping the racks after solder plating will minimize this.
Medium-current dummying at 1.5–2.0 A/dm2 (15–20 asf) will remove tin.
Antimony and bismuth will co-deposit if they are in the 20–100 ppm range, causing brittle deposits. Antimony is usually introduced as an impurity in the copper anodes. It can be removed by low-current-density dummying. Aluminum in amounts greater than 50 ppm may cause dullness in recesses.
Troubleshooting, purification. Acid copper sulfate baths are easy to maintain. Use quantitative analysis to control copper, sulfuric acid and chloride. Add proprietary addition agents, which control brightness, ductility, and leveling, on the basis of amp-hr. You can also regulate their addition by using Hull cells and a copper analysis.
Even though acid copper baths are very tolerant to contamination, they must be purified occasionally. Organic contamination, recognized by a green tint to the solution, is probably the most common, since it is easily introduced into the bath from cleaners, oils, greases, brightener-breakdown products and brightener overloads. Most proprietary systems can tolerate an overdose of additives for a short time but if overdosing continues a light carbon treatment might be necessary.
Pack the filter with activated carbon on non-cellulose filter aid and circulate the solution through the carbon for about three or four turnovers. This should remove enough organics to permit the bath to continue to operate satisfactorily.
Most acid copper baths will eventually require a carbon/hydrogen peroxide treatment to remove organics that cannot be removed by a light carbon treatment. The organic contaminants will cause the bath to have a narrow bright plating range and to produce a dull and burned copper deposit.
Acid Fluoborate Copper Processes
Copper fluoborate is much more soluble than copper sulfate. Therefore, for high-current-density and high-speed processes, the copper ion concentration can be more than double that obtainable in sulfate baths. The fluoborate ion also helps provide solubility and conductivity. But, when compared with sulfate baths, the fluoborate bath has less throwing power.
The copper sulfate bath is more widely used than the fluoborate because it is less expensive. Also, many more additive systems have been developed for copper-sulfate formulations. Copper fluoborate baths are more hazardous to use and harder to waste treat than sulfate baths. And, as with the sulfate baths, the fluoborate solutions are very corrosive, making careful design of plating equipment important.
Chemistry of copper fluoborate baths. If the fluoboric acid concentration is too low (pH above 1.7), the deposit may be dull, dark and brittle. Add boric acid to stabilize the bath and to prevent the decomposition of the fluoborate to fluoride. The anode film and its care in a fluoborate bath are very similar to the requirements described for the copper sulfate bath.
Contamination, purification. Lead is the only metallic impurity known to interfere with the deposition of ductile copper from a fluoborate bath. Addition of small amounts of sulfuric acid will remove the lead by precipitation.
Organic impurities originating from the decomposition of addition agents, solution drag-in or the leaching of tank linings and racks can embrittle the copper deposit and affect the deposit’s appearance and mechanical properties.
A batch carbon treatment followed by filtration or continuous carbon filtration will remove most organics from the bath.
Pyrophosphate Copper
The main use of pyro copper is plating of printed circuit boards. The bath has good throwing power, obtaining surface-to-hole ratios of 1:1 on many PC boards. Some federal agencies specify pyro copper where ductility of the deposit is very important. It is also used for general plating, electroforming and plating on plastics. Even here, though, sulfate acid copper has the largest market share.
Pyrophosphate copper baths are mildly alkaline, making them less corrosive than acid baths. They are essentially non-toxic. They are easy to waste treat, but the phosphate ion is considered a controlled pollutant in some regions. In these cases, controlled waste treatment is required.
Specially formulated pyrophosphate baths can be used for copper strikes on steel and zincated aluminum. Immersion coatings may form on zinc die castings thus cyanide copper strikes are typically used.
Chemistry of pyrophosphate paths. Copper pyrophosphate, Cu2P2O7•3H2O, dissolved in potassium pyrophosphate, K4P2O7 solution, forms the stable complex ion Cu(P2O7)26- from which copper plates. Potassium is used instead of sodium because it is more soluble and has a higher electrical conductivity. Any pyrophosphate in excess of this ratio is called “free” pyrophosphate. Free pyrophosphate is essential for the operation of the bath, providing conductivity and anode corrosion.
The presence of nitrate increases maximum allowable current density and reduces cathode polarization. The use of ammonium nitrate improves the quality of the deposit over that obtained if potassium nitrate is used. Ammonium is added on a regular basis to produce uniform and lustrous deposits and to improve anode corrosion. Excess ammonium can cause copper (I) to form, which hinders adhesion, but ammonium is easily lost by evaporation. Oxalate is a buffer.
Operation. Copper pyrophosphate baths are difficult to control. They require more control and maintenance than cyanide and acid-copper baths.
Orthophosphate. Orthophosphate (HPO42-), which is formed by the hydrolysis of pyrophosphate, promotes anode corrosion and acts as a buffer. At about 100 g/L of orthophosphate, the conductivity and bright plating range of the bath decrease and it produces banded deposits.
A pH below 7.0, a high P2O7:Cu ratio and a bath temperature greater than 60°C (140°F) will increase the formation of orthophosphate. Orthophosphate cannot be chemically removed from pyrophosphate solutions. Therefore, some or all of the bath must be discarded to reduce its concentration.
For printed-circuit-board plating, orthophosphate concentration should not exceed 40-60 g/L. The bath will lose its throwing power and the deposit will be less ductile.
pH. A low pH will precipitate copper pyrophosphate and decrease the throwing power of the bath. A high pH will precipitate copper hydroxide, and anode corrosion will decrease. Potassium hydroxide and pyrophosphoric acid are used for pH adjustments.
Current density. The cathode current den-sity is a function of operating temperature and agitation. The typical range is between 1–9 A/dm2 (10–90 asf). Throwing power and current efficiency drop sharply with increasing current density.
Anode current density is fairly critical and should be kept between 2–4 A/dm2 (20–40 asf). If it is too high, an insoluble oxide tends to form. If too low, the anode efficiency exceeds 100%, causing a buildup of copper in the bath. Permissible current density can be increased by ultrasonic agitation. Current interruption, current reversal or increasing the copper concentration also will increase the permissible current density range.
Agitation. Agitate vigorously to maintain deposit brightness and uniformity. Without agitation, the electrodeposit is brownish and the operating current density is drastically lowered. Air, eductors, mechanical or ultrasonic agitation can be used alone or in combination.
Contamination, purification. The key to successful operation of a copper pyrophosphate solution is careful control of the additives. The solutions are considerably more sensitive than acid copper solutions to organic contamination such as oils, cleaning and buffing compounds, organics leached out of PC boards and breakdown products of organic addition agents.
Organic, cyanide and lead contamination can cause dull, streaked deposits and produce a lower effective current density range. Filtering through sulfur-free plating-grade carbon will remove most organic contamination. Treating with hydrogen peroxide or potassium permanganate before carbon treatment will remove cyanide and severe organic contamination.
Dummying will remove lead and copper. Even on solution makeup, the bath should be dummied at 0.3–0.5 A/dm2 (3–5 asf) to remove metallic impurities. Sodium bisulfite will reduce hexavalent chromium and allow precipitation as chromium hydroxide. Zinc will produce brassy looking deposits and should be dummied out at medium-to-low current density.
Troubleshooting. Chemical analysis for copper, pyrophosphate and ammonia is the starting point for good bath operation. The Hull cell can be used to evaluate additives.
Related Content
Alkaline Cleaning Guide
Gregg Sanko, Senior Chemist, Oakite Products, Inc. provides an overview of the alkaline cleaning process.
Read MoreUnderstanding and Managing White Spots on Anodized Aluminum
Having trouble with spotting defects when anodizing? Taj Patel of Techevon LLC offers a helpful overview of the various causes of white spots and potential solutions.
Read MoreAn Overview of Electroless Nickel Plating
By definition, electroless plating is metal deposition by a controlled chemical reaction.
Read MoreRead Next
Barrel Plating Blues
The following are barrel plating problems that have come up in the recent past...
Read MoreMasking Solutions for Medical Applications
According to Custom Fabricating and Supplies, a cleanroom is ideal for converting, die cutting, laminating, slitting, packaging and assembly of medical-grade products.
Read MoreDelivering Increased Benefits to Greenhouse Films
Baystar's Borstar technology is helping customers deliver better, more reliable production methods to greenhouse agriculture.
Read More