NASF/AESF Foundation Research Project #123: Electrochemical Manufacturing for Energy Applications – 3rd Quarterly Report - Part II
The NASF-AESF Foundation Research Board has selected a project on electrodeposition toward developing low-cost and scalable manufacturing processes for hydrogen fuel cells and electrolysis cells for clean transportation and distributed power applications. This third quarterly report consists of Part II of a review paper, prepared and submitted to a peer-reviewed journal for publication, covering opportunities and challenges for additive manufacturing technologies. Part I, covering the literature on additive manufacturing of SOFCs and SOECs, was published in December 2022.
by
Majid Minary Jolandan*
Department of Mechanical Engineering
The University of Texas at Dallas
Richardson, Texas, USA
A Note from the AESF Foundation Research Board
During this July-September quarter, Dr. Minary moved to the University of Texas at Dallas (UTD). Accordingly, this report covers a condensed period from July 1 to August 8, 2022.
As is standard practice with academic contracts, the grant will follow the awarded professor (Dr. Minary). Logistically, there has been an offset in the program with no work was performed from August 8 to October 1, 2022. On Oct 1, UTD initiated the grant, and the work will continue and proceed for the next three years and one quarter, pending subsequent board approval for each additional year.
Accomplishments during the quarter
During the transition period, Dr. Minary’s time has been spent in establishing his new laboratory at UTD and all the work that is involved with layout, construction and acquiring new equipment. However, a review was prepared and submitted to the peer-reviewed Ceramics Journal for publication. What follows here is Part II of the review paper. Part I was published in December 2022 and a printable PDF version of Part I is available by clicking HERE; a PDF of Part 2 is available HERE.

Formidable Challenges in Additive Manufacturing of Solid Oxide Electrolyzers (SOECs) and Solid Oxide Fuel Cells (SOFCs) for Electrolytic Hydrogen Economy toward Global Decarbonization – Part II
by
Majid Minary Jolandan
Department of Mechanical Engineering
The University of Texas at Dallas
Richardson, Texas, USA
ABSTRACT
Solid oxide electrolysis cells (SOECs) and solid oxide fuel cells (SOFCs) are the leading high temperature devices to realize the global "Hydrogen Economy". These devices are inherently multi-material (ceramic and cermets). They have multi-scale multi-layer configuration (a few microns to hundreds of microns) and different morphology (porosity and densification) requirements for each layer. Adjacent layers should exhibit chemical and thermal compatibility and high temperature mechanical stability. Added to that is the need to stack many cells to produce reasonable power. The most critical barriers to global widespread adoption of these devices have been their high cost and issues with their reliability and durability. Given their complex structure and stringent requirements, additive manufacturing (AM) has been proposed as a possible technological path to enable low-cost production of durable devices to achieve economies of scale. However, currently, there is no single AM technology capable of 3D printing these devices at the complete cell level, or even more difficult at the stack level. Part II of this article provides an overview of opportunities and challenges for AM to be a viable path for manufacturing of SOECs and SOFCs. A list of recommendations is provided to facilitate such efforts.
Keywords: SOEC, SOFC, hydrogen economy, renewable energy, decarbonization, additive manufacturing, market competitiveness, scale-up and high-volume manufacturing.
4. Opportunities and challenges for AM technologies
Considering the reported AM work in the literature, limitations of the current manufacturing technologies, several challenges that must be overcome for AM to be a viable path for manufacturing of SOECs and SOFCs are outlined below. Corresponding recommendations are provided to facilitate such efforts.
4.1 Eliminate or reduce stacking and lamination steps and enhance durability:
Current AM technologies can print individual components such as the electrolyte or anode/cathode, and in some cases, several of the components such as anode and electrolyte. However, for AM technologies to compete with traditional manufacturing technologies for SOECs and SOFCs market, these technologies should eliminate or reduce the stacking steps, and/or enable continuous printing of the integrated layers at the cell level and/or stack level, something that remains a formidable challenge.1 Reducing the number of parts and interfaces will result in minimizing degradation and failure opportunities in these devices. Another point of view may be using AM only for components that are suitable for 3D printing, rather than printing integrated cells and stacks. In this approach, a combination of additive and traditional manufacturing methods maybe used. Essentially, AM will be used only if it can deliver reliable component(s) with complex geometries that cannot be manufactured otherwise, and when there is enough performance gain justification. An example would be printing the electrolyte using DLP/SL and adding the electrodes by spraying or brushing; or printing the electrolyte using an inkjet printer on a cathode fabricated by a traditional route such as power consolidation.
The push toward lower cost hydrogen should be achieved with a long-term consideration of durability and efficiency. Such efforts, particularly in relevance to AM technologies, demand a holistic approach integrating standardization of testing and characterization protocols (including accelerated stress tests, ASTs), identification of common degradation mechanisms (preferably over the entire device lifetime and under realistic operation conditions) and their mitigation strategies, and improving durability (at the cell and stack level) and overall efficiency. Reproducible and uniform manufacturing and performance for high-volume manufacturing is a requirement for quality assurance and quality control (QA/QC). Currently, there are no studies in the literature on the long-term performance, durability and degradation mechanisms of 3D printed SOECs and SOFCs.
4.2. Facilitate co‐sintering
Heat treatment comprise ~40% of the total manufacturing cost of these electrochemical devices (due to high temperature and long duration sintering and expensive equipment), and hence it is a major area for cost reduction.2 This cost reduction is mostly through co-firing of multiple layers. Several of the limitations or challenges of AM are common with the traditional fabrication methods. An example is the requirement for co-sintering (co-firing) multiple layers of different materials (sometime metals and ceramics) with different sintering temperature and time requirements. Heating and cooling of such complex multilayer, multi-material parts is further complicated by the different CTE of adjacent layers. Continuous sintering (as opposed to batch furnaces) is considered as a cost reduction strategy. Similarly, microwave and plasma-assisted sintering are also considered as viable cost reduction strategies.3 AM may enable denser and thinner layers, which will result in lower heat treatment time and cost, and more sample-to-sample uniformity. In addition to materials, another consideration in co-sintering is the influence of pore-formers on the shrinkage and sintering characteristics of electrodes.4 Co-sintering is particularly attractive when electrodes are fabricated by catalyst infiltration into a porous ceramic scaffold, since the interface between the ceramic electrolyte and porous ceramic electrode will be strong, or no interface will be formed if the processing method can fabricate the porous electrode and dense electrolyte continuously. AM may also facilitate manufacturing of functionally graded structures such that transition from one material to another is optimized in terms of CTE.
4.3. Enable lower temperature operation
Potential AM technologies should also address the current push toward lower temperature operation (to ~ 500-600°C or lower). Lower temperature operation is desirable to reduce or resolve some of the challenges such as thermal management and the need for insulation, thermal stress, high degradation rate of current collectors and sealings. Lower operation temperatures may result in an increase in the device efficiency, as well as possibility of using metals and polymers for these devices. This trend either requires thinner layers (using current materials) to reduce ionic/electronic resistance or discovery of new low temperature oxygen ions conductors. Additionally, microstructural variations such as columnar grains that have less resistance, substitutional doping and defect control, and fabrication of interfaces with less resistance (or elimination of interfaces in monolithically printed devices) may facilitate lower temperature operations.
The trend toward low temperature and thinner layers (tens of microns) will require printing technologies with better resolution. Thickness of each layer in DLP/SL process is ~50 μm, while to reduce the resistance, lower thickness electrolyte is required. One way to achieve thinner layers using AM is incorporating (printing) topologically optimized mechanical supports, for example an electrolyte with thin and thick sections which requires complex 3D designs.5
4.4. Enable high‐volume production and lower total cost
Ultimately, AM technologies will be compared to high-throughput processes such as roll-to-roll process. Hence, the cost analysis is a major factor for the success of AM technologies. For these electrochemical devices, materials and manufacturing costs are the major portion (~80%) of the total cost.2 By increasing the number of units per year, the total manufacturing cost will significantly decrease. Currently, SOEC systems are not at cost-parity with conventional hydrogen production technologies (e.g., natural gas reforming) and will require technology development to achieve widespread deployment and commercialization. Likewise, SOFCs will require significant technology advancement to be cost-competitive with conventional combustion-based technologies currently used in stationary and vehicular applications. Without further development, these technologies are unlikely to economically support the predicted hydrogen market size.
There are three factors in cost reduction of clean electrolytic H2, namely electricity, capital cost and fixed operations and maintenance. The current DOE goal is $2/kg by 2026 and $1/kg by 2031. The key enablers for lower cost electrolytic H2 include low-cost electricity, high electrical efficiency, low-cost capital expense, increased durability and lifetime, low-cost manufacturing processes, manufacturing at MW-scale and increased power density. Analyses have projected a 4.5-6-time reduction in SOEC stack manufacturing cost through increasing manufacturing rates from 25 MW to 1 GW per year.2
We should note that several manufacturing steps of 3D printing and traditional manufacturing are identical or similar depending on which process is used. These include slurry preparation (ball milling, rheological characterization), debinding (binder burnout) and (co)sintering. Accurate cost analysis for 3D printing technologies for production of SOECs and SOFCs requires considerations of the potential economies of scale. With increase in demand, raw materials cost may reduce, and combined with enhanced device efficiency and durability, and increased manufacturing throughput, may result in an overall cost reduction and economic competency of 3D printing.
As an example, interconnects are often a major contributor to the total cost. Since interconnects are exposed to both oxidizing and reducing high temperature environments, ceramic materials can offer a stable and reliable option, albeit at a steep price. Metal based interconnects will be more promising as lower temperature range (600-800°C) devices are developed. Additive manufacturing can offer solutions for both metallic and ceramic interconnects, particularly for novel designs that incorporate optimized gas channels, and better mechanical performance.
4.5. Enable complex geometries (beyond planar and tubular cells), different cell configurations and larger cell size
Currently the most common geometries are planar and tubular geometries. Each of these designs have advantages/disadvantages in terms of volumetric efficiency, startup time, sealing quality and reliability, and manufacturing cost.6 Modified planar designs enabled by AM may incorporate a wave-like (corrugated) structure or micropillar covered surface to increase the surface area. Additionally, AM may enable incorporation of optimized gas flow channels within the cell structure to improve transport, and ultimately result in higher power density. On the other hand, for more complex geometries, the dimensional changes during binder burnout and sintering become more challenging to predict and account for, which will require sophisticated modeling and simulation.
Generally, there are three configurations for each cell that include electrolyte-supported, electrode-supported, and metal-supported and each may have their own subcategories.7,8 For example, an anode-supported cell can be monolithic, in which the entire anode has the same morphology, or it can be bi-layer, in which two different layers with different morphology and composition form the anode. In a bi-layer configuration, the thinner anode layer closer to the electrolyte is considered as the anode functional layer (AFL). Similarly, the cathode functional layer (CFL) may be implemented on the cathode side. Being able to 3D print these functional layers with fine microstructure and optimized composition is expected to enhance the electrochemical active sites at the electrode/electrolyte interface and reduce the contact resistance.
In metal-supported cells (MS-SOFCs) the Ni-YSZ electrode support is replaced with a porous stainless steel, Ni or other metals. The porous metal support is believed to improve strength (mitigate brittle failure associated with ceramics) and tolerance to aggressive operating conditions such as rapid thermal cycles, increase the redox tolerance, and reduce the cost.8 Currently, metal-supported cells are not as developed as anode-supported cells, and some aspects of degradation and oxidation issues need to be addressed. Given the much-developed AM technologies for metals, development of metal-supported cells may benefit from 3D printing.
Larger cells require less stack materials and supporting infrastructure such as insulation, and hence result in higher power density. Therefore, increase cell size is considered as a pathway for overall cost reduction. However, since density of flaws increases with increase in size, these mostly ceramic devices would suffer from lower strength for larger sizes (Griffith theory of fracture). A similar concept may be applicable to having integrated channels into the 3D printed devices, since they will change the entire stress field and stress concentration points, as well as electrochemical performance. Hence, mechanics and electrochemical performance analysis must be performed for various 3D printed designs.
4.6. Enable morphology (mostly pores) control
Porosity plays a significant role in cell and electrode polarization, hence control and optimization of pore morphology is a key to any manufacturing process. Traditional processing such as tape casting with pore-formers provide limited control over pore morphology. Although an open-pore network is required, orientation and tortuosity of the pores deserve special attention. If the pores are aligned in the direction of the electrolyte/electrode interface, they are ineffective. Additionally, a tortuous path hinders gas diffusion. If AM can provide a type of porosity that is aligned perpendicular to the electrolyte/electrode interface (for example cylindrical), the generated porosity will effectively enhance gas diffusion. Creative channels and pores can be designed, for example the ones inspired by biological systems that optimize the gas diffusion, while maintaining the mechanical integrity. We note that aspects of pore engineering vary depending on the cell configuration. If the structural support in the cell is provided by anode (anode-supported cells), the thickness of anode needs to be higher (0.5-1.5 mm). With such a high thickness, engineering of pore morphology becomes more important to prevent increased polarization.4
4.7. Enable “true” multi‐material, multiscale 3D printing
The “multi-material printing” term has been used in various context in the literature,9 and it may need clarification to be accurate. A printer that can print one of the general family of materials (polymers, or metals, or ceramics) in various forms and compositions may be called a multi-material printer. Examples may include a polymer printer that can print two different polymers with different stiffnesses, or colors, as in FDM printers; or a multi-nozzle printer that is connected to two or more reservoirs and can print each or combination of the inks by mixing and extrusion through the same nozzle such as in direct write-type printers; or a metal printer that can print alloys with different composition such as in direct energy deposition printers.
The case for printing SOECs and SOFCs goes much beyond these points and can be argued that currently there is no such technology to satisfy all the requirements. A hypothetical multi-material multi-scale printer to print these devices should be able to print ceramics (electrolyte), cermets (anode and cathode), and metals (contacts and interconnects), in addition to glass sealings. The printer should be able print at multiple scales, as thin as a few microns (for electrolyte, and anode/cathode functional layers) and several hundreds of microns (for example for anode support). One current inherent limitation of AM technologies is generation of porous structures required for electrodes, because these methods are not compatible with pore-formers (nozzle clogging in inkjet printer and light scattering in DLP and SL). Therefore, the ideal printer should be able to adjust and control the porosity level (porous for anode and cathode and dense for electrolyte). Such requirements are nontrivial.
If manufacturing of complete SOFCs/SOECs is intended, SL and DLP have limitations in achieving multi-material printing capability. To print multi-materials in a DLP/SL type printer, multiple vats with different resin compositions (multiple exchanging resin vats) should be used.9 In this method, the printing plate (platform) must be moved from one bath to another, with an additional step of washing and drying between each vat, which overall results in a slow and complex process, with possible contamination in between different vats and compromised materials composition and possibility of low adhesion between interlayers of different materials in the print.9 Although inkjet printing is inherently compatible with different classes of materials, it is mostly limited to 2D geometries.
4.8. Enable robotic‐assisted multi‐printer process
Developing a printer that can print these devices is a monumental challenge. However, as it is common in the semiconductor industry, for a chip to be manufactured multiple processes using multiple machines are involved. If such processes can be developed that are able print various components of these devices in series, such manufacturing process may be amenable to robotic and automation.10 Given that a single printer is unlikely to print an entire cell or stack, robotic-assisted manufacturing may be relevant for SOFCs and SOECs. Robotic systems can transfer parts from one printer to the next to complete the process. In traditional manufacturing process, tape-casting, heat treatment, and stack assembly are considered the best candidates for automation. Therefore, this may raise this question that “what the advantages of such multiprocess 3D printing would be compared to traditional manufacturing processes, i.e., automation of tape-casting, and stacking process?” A question that needs to be answered.
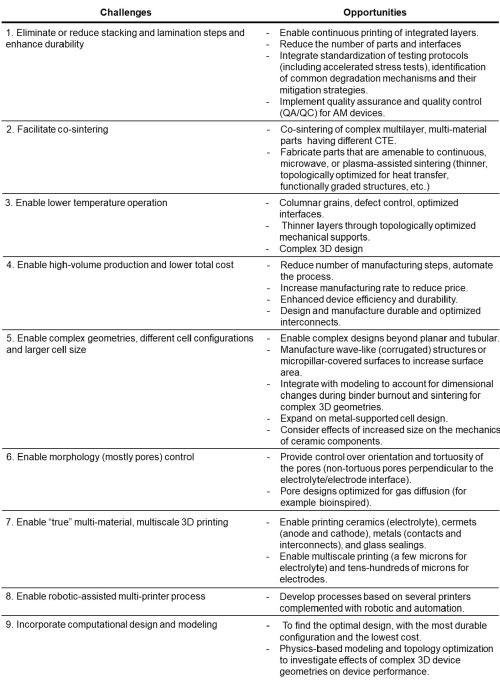
Table 1 - Challenges and opportunities of AM of SOECs and SOFCs.
4.9. Incorporate computational design and modeling
Computational design may play a major role in efforts toward commercialization of these technologies, given the high cost of manufacturing and numerous iterations to find the optimal design with the most durable configuration and the lowest cost. AM should be combined with physics-based modeling and topology optimization to investigate effects of complex 3D device geometries on temperature distribution, flow velocity, pressure, and air and fuel gaseous concentrations through the cell and gas channels, and in turn, the effect of these parameters on the potential and current density distribution and performance of the device. Modeling of these devices often requires multiphysics software platforms, including electrochemistry, fluid flow, heat transfer, thermal and structural models. As an example, it is known that the gas channels in electrodes have paramount effect on oxygen distribution and cell performance.
Table 1 provides a summary of challenges and opportunities of AM of SOECs and SOFCs.
5. Outlook
Overall, it can be argued that AM can provide a viable technological path for at-scale and low-cost manufacturing of SOECs and SOFCs, or thinking more conservatively, it can address several of the outstanding manufacturing issues. However, current AM technologies have major limitations for production of these complex devices, in terms of scale, time, cost, material compatibility, among others. It is expected these issues will be resolved overtime as AM technologies get more matured and new AM technologies are developed. This endeavor will require investments from both the governments and the private sector to organize global collaborative efforts and initiatives.
6. Acknowledgements
This work is supported by the AESF foundation under the AESF Foundation Research Program and the US National Science Foundation (CMMI award number 2152732).
7. References
1. L. Wei, et al., “A novel fabrication of yttria-stabilized-zirconia dense electrolyte for solid oxide fuel cells by 3D printing technique,” International Journal of Hydrogen Energy, 44 (12), 6182-6191 (2019).
2. High Temperature Electrolysis Manufacturing Workshop Summary Report, Hydrogen and Fuel Cell Technologies Office, U.S. Department of Energy, March 2022.
3. M. Bhattacharya and T. Basak, “A review on the susceptor assisted microwave processing of materials,” Energy, 97, 306‐338 (2016).
4. B. Shri Prakash, S. Senthil Kumar and S.T. Aruna, “Properties and development of Ni/YSZ as an anode material in solid oxide fuel cell: A review,” Renewable and Sustainable Energy Reviews, 36, 149-179 (2014).
5. B. Xing, et al., “Dense 8 mol% yttria-stabilized zirconia electrolyte by DLP stereolithography,” Journal of the European Ceramic Society, 40 (4), 1418-1423 (2020).
6. M. Singh, D. Zappa and E. Comini, “Solid oxide fuel cell: Decade of progress, future perspectives and challenges,” International Journal of Hydrogen Energy, 46 (54), 27643‐27674 (2021).
7. M.C. Tucker, “Progress in metal‐supported solid oxide fuel cells: A review,” Journal of Power Sources, 195 (15), 4570‐4582 (2010).
8. M.C. Tucker, “Progress in metal‐supported solid oxide electrolysis cells: A review,” International Journal of Hydrogen Energy, 45 (46), 24203‐24218 (2020).
9. U. Shaukat, E. Rossegger and S. Schlögl, “A Review of Multi‐Material 3D Printing of Functional Materials via Vat Photopolymerization,” Polymers, 14 (12), 2449 (2022).
10. E. MacDonald and R. Wicker, “Multiprocess 3D printing for increasing component functionality,” Science, 353 (6307), aaf2093 (2016).
8. Past project reports
1. Quarter 1 (January-March 2022): Summary: NASF Report in Products Finishing; NASF Surface Technology White Papers, 86 (10), 17 (July 2022); Full paper: http://short.pfonline.com/NASF22Jul1 .
2. Quarter 2 (April-June 2022): Summary: NASF Report in Products Finishing; NASF Surface Technology White Papers, 87 (1), 17 (October 2022); Full paper: http://short.pfonline.com/NASF22Oct2 .
3. Quarter 3 (July-September 2022) Part I: Summary: NASF Report in Products Finishing; NASF Surface Technology White Papers, 87 (3), TBD (December 2022); Full paper: http://short.pfonline.com/NASF22Dec2.
9. About the Principal Investigator for AESF Research Project #R-123
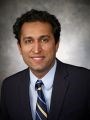
Majid Minary Jolandan is Associate Professor of Mechanical Engineering at The University of Texas at Dallas, in Richardson, Texas, in the Erik Jonsson School of Engineering. His education includes B.S. Sharif University of Technology, Iran (1999-2003), M.S. University of Virginia (2003-2005), Ph.D. University of Illinois at Urbana-Champaign (2006-2010) as well as Postdoctoral fellow, Northwestern University (2010-2012). From 2012-2021, he held various academic positions at The University of Texas at Dallas (UTD) and joined the Faculty at Arizona State University in August 2021. In September 2022, he returned to at UTD as Associate Professor of Mechanical Engineering. His research interests include additive manufacturing, advanced manufacturing and materials processing.
Early in his career, he received the Young Investigator Research Program grant from the Air Force Office of Scientific Research to design high-performance materials inspired by bone that can reinforce itself under high stress. This critical research can be used for aircraft and other defense applications, but also elucidate the understanding of bone diseases like osteoporosis.
In 2016, he earned the Junior Faculty Research Award as an Assistant Professor at the University of Texas-Dallas – Erik Jonsson School of Engineering.
* Corresponding author:
Dr. Majid Minary Jolandan
Department of Mechanical Engineering
The University of Texas at Dallas
800 West Campbell Road
Richardson, TX 75080-3021
Office: ECSW 4.355H
Phone: 972-883-4641
Email: majid.minary@utdallas.edu
Related Content
NASF/AESF Foundation Research Project #123: Electrochemical Manufacturing for Energy Applications - 6th Quarter Report
The NASF-AESF Foundation Research Board selected a project on electrodeposition toward developing low-cost and scalable manufacturing processes for hydrogen fuel cells and electrolysis cells for clean transportation and distributed power applications. In this period, work focused on 3D printing anode support for solid oxide fuel cells, SOFC (or cathode for solid oxide electrolyzers, SOEC) based on our designed optimization outlined in the previous report.
Read MoreNASF/AESF Foundation Research Project #123: Electrochemical Manufacturing for Energy Applications – 8th Quarterly Report
The NASF-AESF Foundation Research Board selected a project on electrodeposition toward developing low-cost and scalable manufacturing processes for hydrogen fuel cells and electrolysis cells for clean transportation and distributed power applications. This report covers the 8th quarter of work (October-December 2023, continuing work on 3D printing anode support for solid oxide fuel cells and electrolyzers. Work involved the effect of sintering temperature on the amount of porosity and grain size in 3D printed yttria-stabilized zirconia (YSZ).
Read MorePossibilities From Electroplating 3D Printed Plastic Parts
Adding layers of nickel or copper to 3D printed polymer can impart desired properties such as electrical conductivity, EMI shielding, abrasion resistance and improved strength — approaching and even exceeding 3D printed metal, according to RePliForm.
Read MoreNASF/AESF Foundation Research Project #123: Electrochemical Manufacturing for Energy Applications - 7th Quarterly Report
The NASF-AESF Foundation Research Board selected a project on electrodeposition toward developing low-cost and scalable manufacturing processes for hydrogen fuel cells and electrolysis cells for clean transportation and distributed power applications. In this period, we followed our work on 3D printing anode support for solid oxide fuel cells, SOFC (or cathode for solid oxide electrolyzers, SOEC) based on our designed optimization outlined in the previous report. We worked on optimizing the printing parameters, obtaining binder burn out and sintering profiles to obtain printed parts with desired geometry and properties.
Read MoreRead Next
A ‘Clean’ Agenda Offers Unique Presentations in Chicago
The 2024 Parts Cleaning Conference, co-located with the International Manufacturing Technology Show, includes presentations by several speakers who are new to the conference and topics that have not been covered in past editions of this event.
Read MoreDelivering Increased Benefits to Greenhouse Films
Baystar's Borstar technology is helping customers deliver better, more reliable production methods to greenhouse agriculture.
Read MoreEducation Bringing Cleaning to Machining
Debuting new speakers and cleaning technology content during this half-day workshop co-located with IMTS 2024.
Read More