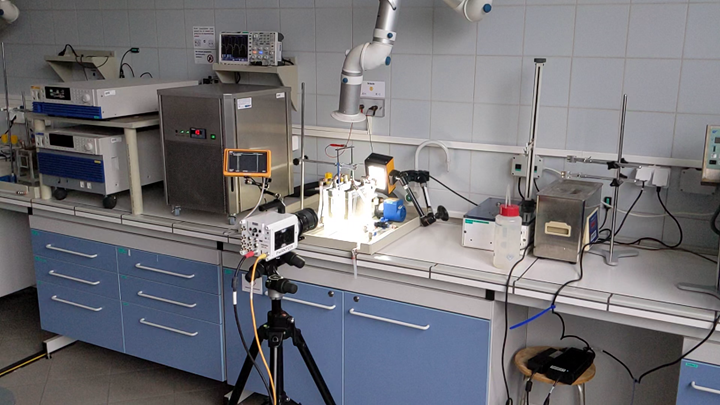
This workstation was designed to observe the PEO electrochemical surface treatment process.
Photo Credit: All images courtesy of T2 Marketing Communications
An extension of traditional anodizing for treating metal surfaces, plasma electrolytic oxidation (PEO) involves exposing a metal workpiece to high voltages to improve the part’s wear properties. Part of the process is the formation of micro-discharges that occur in microseconds on the metal’s surface as it becomes conductive — similar to what happens during a thunderstorm. Like tiny sparks of lightning, these micro-discharges require some of the fastest, most light-sensitive imaging tools to capture and analyze.
What is PEO?
PEO is an electrochemical surface treatment for processing metals like aluminum, magnesium and titanium to improve the metal surface’s wear and corrosion resistance. Like traditional anodizing, PEO involves placing a metal workpiece — in this case, an anode — into an electrolyte bath with a counter-electrode, or cathode, and then closing the electrical circuit. The electrochemical reactions that occur on the treated metal surface cause the material to oxidize and generate a coating.
“The film makes the material harder and more durable and corrosion-resistant,” explains Maciej Sowa, PhD, who heads the research at Poland’s Silesian University of Technology. “The treated metal is used in many smartphone cases, as well as for heat shields in aerospace applications.”
What differentiates PEO from traditional anodizing is its wider range of voltages. According to Sowa, traditional anodizing typically relies on voltages less than 120 volts (V), while PEO uses voltages as high as 700 V. The growth of the oxide film increases the voltage across the coating. “Eventually, the electric field becomes so high, it exceeds the dielectric breakdown potential of the oxide film, creating electrostatic micro-discharges that result in localized plasma reactions,” Sowa says. The presence of the plasma causes the oxide film to grow further, giving rise to the unique properties of PEO oxide coatings.
“Exposing the submerged workpiece to higher voltages causes the metal surface to grow in thickness,” Sowa says. “This process causes microscale discharges on the surface as it suddenly becomes conductive — like what happens during a thunderstorm.”
To help Sowa and his team observe the formation and progression of these micro-discharges, they recorded their experiments using a Phantom T3610 high-speed camera, which uses back side illumination (BSI) sensor technology to maximize performance and light sensitivity for sub-microsecond exposure times. Given the very fast, microscale nature of the PEO process, this camera proved to be the perfect tool for Sowa’s analysis, which is part of a much larger project aimed at positioning PEO as an environmentally friendly technology (see sidebar below). “The process is still a relatively young technology, requiring research to overcome its scientific and technical issues before it can develop on a larger scale,” Sowa says.
The Experimental Setup
Sowa and his team ran their PEO experiments using 6061 aluminum alloy, cut into blocks measuring 10 x 10 x 20 millimeters, in an asymmetric AC regime, where the peak anodic and cathodic voltages were 430 V and 100 V, respectively. They initially set the frequency of the voltage fluctuations to 500 Hertz (Hz) and then lowered it to 100 Hz. In the positive (anodic) half-cycle, surface micro-discharges developed and were visible in the form of small sparks. Then, in the negative (cathodic) half-cycle, these sparks were quenched to prevent the coating from overheating and developing thermal cracks.
In addition, the researchers fitted the electrolyzer — the bath made of poly(methyl methacrylate) (PMMA) — with a square, quartz window to observe the specimens during the PEO process. “The quartz allowed ultraviolet light to pass through the window, enabling us to analyze the UV visible light spectrum with the camera,” Sowa explains.
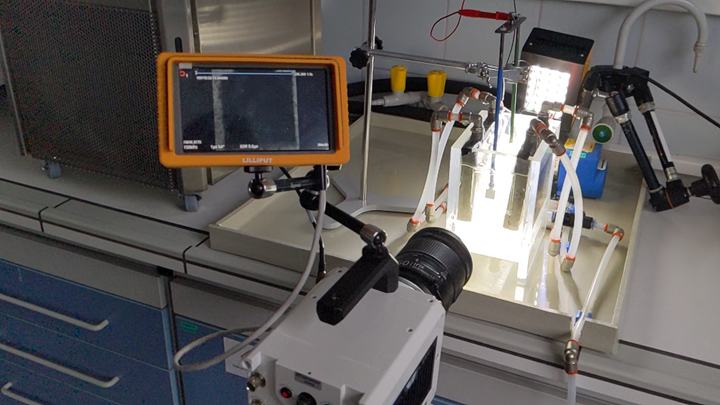
The experimental setup.
The team used the T3610 camera to record the oxidation process at 200,000 frames per second (fps), with an exposure time of 5 microseconds (µs) and spatial resolution of 512 x 256 pixels. “The camera helped us gather information about the longevity of the micro-discharges, which usually occur in the 50 to 100 microsecond range, as well as the surface density of the plasma over time,” Sowa says.
“Understanding these phenomena, including the nature and progression of the micro-discharges, can help scientists who use PEO to adjust process parameters in order to smooth out the plasma behavior and limit the destructive nature of the sparks,” Sowa adds. These parameters can include frequency, current density and electrolyte composition.
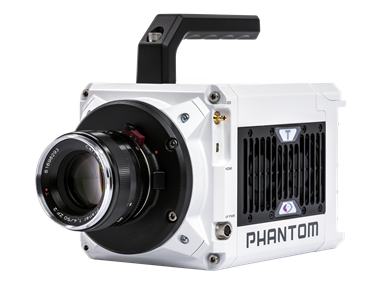
Phantom T3610 camera
A Lightning-Fast Tool for a Lightning-Fast Application
According to Sowa, the Phantom T3610 high-speed camera was indispensable to the PEO experiments. At full 1280 x 800 resolution, it can capture over 38,000 fps, maintaining 38 Gpx/s throughput, and tops out at 875,000 fps at reduced resolutions. The T3610 also offers sub-microsecond exposure times — 1 µs standard and 190 nanoseconds (ns) with the export-controlled FAST option. These exposure times make the camera an ideal tool for extremely fast applications like PEO, which involve capturing the micro-discharges over a few microseconds.
“In addition, whenever you jump to high frame rates — in our case, 200,000 fps — you lose a lot of spatial resolution,” Sowa says. “The Phantom camera did an exceptional job balancing both and providing us with high-quality images. Compared to previous experiments involving high-speed cameras, I could see significantly more detail.”
Driving the T3610’s speed, throughput and light sensitivity is its innovative BSI sensor. This technology increases the pixel surface area that can capture photons, balancing frame rate, resolution and sensitivity like never before. Whereas traditional front side illuminated (FSI) architectures have circuitry that prevents some incident light from reaching the pixels, BSI sensors provide a direct route for light to reach the light-receiving surface, making it possible to capture extremely fast, microsecond events without motion blur.
Other features of the T3610 that helped Sowa and his team capture highly detailed images of the PEO process include the following:
- Lab-friendly housing. The T3610 naturally complements the space-limiting nature of many laboratories, featuring a compact housing that is 50% lighter than equivalent camera models. Despite the smaller size, however, the camera is exceptionally durable, featuring active cooling capabilities. In fact, at the time the researchers performed these experiments, Poland was hit with a massive heat wave. “A lot of our other equipment in the lab failed, but the camera did not,” Sowa says.
- User-friendly software. The T3610, like all Phantom high-speed cameras, works with Phantom Camera Control (PCC) software, which features many advanced features, such as continuous recording for automated workflows, as well as imaging tools like crop, resample, tone curves, filters and more. “The software was very intuitive,” Sowa says. “It also offered tutorials with helpful hints on how to use the camera most effectively.”
- Focus assist. According to Sowa, capturing the sparking process was “tricky” at times, as a lot of gas generated during the process obscured his view. Fortunately, the PCC software comes with a focus assist feature that helps users clearly visualize when details in the image come into focus, facilitating the recording process. “In addition, we were recording a dangerous, high-voltage process,” Sowa says. “Our ability to use a PC to adjust the focus meant we never had to move the camera or samples around.”
Understanding the Soft-Spark Cascades
According to Sowa, the T3610 enabled him to see how the PEO oxidation process progressed over time — something that would not have been possible otherwise. “Without the camera, I would have had to use a microscope to view the end result of the process, after the fact, rather than the evolution of the process itself.”
In addition to observing the process, Sowa and his colleagues gained insights into the nature of the sparks themselves. Over the course of their experiments, they observed the dynamics of surface plasma micro-discharges in what Sowa calls the “soft-sparking regime,” which is the state of the treatment where the micro-discharges diminish in size and acoustic emission intensity. To achieve this state, the team drastically reduced the treatment voltage from around 425 volts to below 360 volts, which decreased how much energy the process was consuming.
“Soft sparking increases the growth rate of the oxide film, making it possible to form corundum, which is a crystalline form of aluminum oxide, further improving the wear resistance of the modified surface,” Sowa says. “So far, there are no studies like this in the literature. There are only those devoted to hard sparks — not this softened variant.”
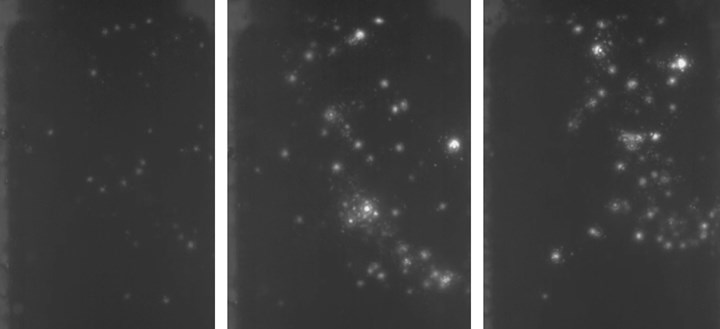
Observing the soft-spark cascades during PEO.
Sowa believes the lack of studies on soft sparks is due to the fact that these lightning-fast sparks are difficult to control. According to him, soft sparks quickly develop into cascades, which are clusters of sparks that occur in the same spot many times in a row. “Ideally, we want to have as little cascading as possible. We’re trying to determine the conditions that reduce the amount of cascading so that we have individual sparks instead, enabling us to limit the potential damage of the sparks and create more uniform, robust and cost-effective coatings.”
Next Steps
Building on this research, Sowa’s next steps include studying PEO to draw causal conclusions. “For example, we want to understand and characterize specific micro-discharge behavior and the kind of surface it produces. That’s what we want to do next.”
These PEO experiments are part of a larger project financed by the National Center for Research and Development (NCRD) in Poland called “Development of technology for plasma electrolytic formation of anodic oxide coatings on light metal alloys towards comprehensive surface protection systems” (project no. LIDER/30/0116/L-11/19/NCBR/2020; realization period: 2021-2024). The project plans to:
- Determine the effect of treatment conditions on the structural and functional characteristics of anodic oxide coatings obtained in the PEO process.
- Demonstrate the effect of additional pre-treatments on the quality of PEO layers.
- Explore the possibility of adding functionalities to the layers by surface finishing.
- Develop a method prototype to implement and prepare the technology for commercialization.
About the Author
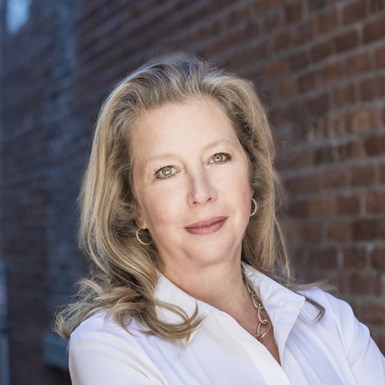
Victoria Case
Victoria Case is the director of product management and marketing for AMETEK Vision Research.
Related Content
An Overview of Electroless Nickel Plating
By definition, electroless plating is metal deposition by a controlled chemical reaction.
Read MoreHow to Address Declining Powder Coating Coverage Over Time
Fine particles from reclaim could be to blame for powder coating problems that emerge over time. Avoid problems by keeping hooks clean, maintaining guns and using reclaim powder quickly to avoid accumulation of fines.
Read MoreZinc Phosphate: Questions and Answers
Our experts share specific questions about zinc phosphate and pretreatment
Read MoreHow to Choose Between Sulfate and Chloride-Based Trivalent Chromium
There are several factors to consider when choosing between sulfate and chloride-based baths for trivalent chromium plating. Mark Schario of Columbia Chemical discusses the differences and what platers should keep in mind when evaluating options.
Read MoreRead Next
Understanding and Managing White Spots on Anodized Aluminum
Having trouble with spotting defects when anodizing? Taj Patel of Techevon LLC offers a helpful overview of the various causes of white spots and potential solutions.
Read MoreCrazing and Cracking in Anodizing
Understanding the differences in cracking and crazing in anodic coatings, and insights for mitigating defects in anodized parts.
Read MorePEO coatings sparking new interest
Plasma electrolytic oxide surface treatment for aluminum, magnesium or titanium
Read More